“The sun became dark and its darkness lasted for one and a half years… Each day it shone for about four hours and still this light was only a feeble shadow… the fruits did not ripen and the wine tasted like sour grapes.” Michael the Syrian, about a 6th century eruption
It smells. Sulfur is among the easiest detectable substances, and for good reason. It is a sign of decay, as in rotten eggs, and it is actively damaging to our bodies. Although not infallible, our noses give us a powerful stimulus to stay away from it. It also helps us to avoid active volcanoes. The sulfur smell is a good indicator of a potential eruption – and it can kill well before the lava does. But that gets ahead of the story.
The sulfur in our air comes from three main sources. The first is the living world: biogenic sulfur. Particular types of bacteria live on it, and many people guts have plenty of these (it actually depends on your diet). Life puts some 25 Tg sulfur per year into the atmosphere. (Tg stands for teragram, or 1012 gram, or about 1 billion kilogram, or 1 megaton.) (The word ‘tera’ may well come for Thera, the big eruption which devastated the pre-Greek Mediterranean. Volcanoes show up everywhere.) The next big source is humanity, in its hugely successful enterprise to change the world using coal and oil. We produce about 80 Tg per year by burning fossil fuels. Finally, volcanoes produce on average 9 Tg per year. About a third of the volcanic emissions come from non-erupting volcanoes, and the rest is associated with eruptions or with continuously active lava lakes. A big eruption can increase the number substantially in a particular year.
These numbers suggest that volcanoes are not a big problem. But it depends on where you look. Human (anthropogenic) emissions occur near the surface, where our chimneys and exhausts are. As a result our sulfur stays at low altitudes, and comes out of the atmosphere quite quickly; the majority does not travel far. Volcanoes deposit their gasses higher in the troposphere, by ejecting them at high temperatures and with a considerable push. Once they are in the upper atmosphere , they remain airborne for longer. At ground level, humanity is worse (you do not want to live close to a coal burning power station), but higher up, volcanoes have a greater effect because their sulfur hangs around and travels further.
Volcanoes produce sulfur gas mainly as SO2, with minor amounts of H2S and H2SO4 and traces of carbonyl sulfide (COS) and its precursor carbon disulfide (CS2). In the air, H2S quickly oxidises to SO2. Underground, SO2 is not very volatile and it prefers to stay in the magma until the last possible moment. CO2 comes out of the magma while it still kilometers deep, and finds its way to the surface long before the magma does. Therefore CO2 is mainly produced by non-erupting volcanoes, and comes up all over the place, perhaps far from the eventual exit point of the magma. SO2 is a far more reluctant gas. When you find it in the air near a volcano, magma must be close to the surface and lava may follow soon. SO2 signals lava: it indicates an open vent. In Kilauea, the SO2 came from the caldera, and from the erupting fissures. The one exception is when fumaroles bring up sulfur through circulating water, exchanging gas with the magma. The rocks around these fumaroles can build up the characteristic yellow deposit.
Earthly sulfur
Sulfur is suprisingly common, but well hidden and not easy to see. There are many sulfur minerals in rocks (such as pyrite, or FeS), and the ocean has a considerable amount of sulfate. Epsom salts contain magnesium-sulfide. A common sulfate mineral is gypsum, used for alabaster, plaster, and chalk. Gypsum comes in part from sedimentation in volcanic hot springs, showing that volcanoes have an important role in the sulfur cycle. In he bible, sulfur was known as brimstone, found around the Dead Sea where it too comes up from the deep.
But in spite of it being the 5th most common element on Earth, sulfur is almost absent in the atmosphere. SO4, the most common form, is highly solvable and loves to be in water. The atmosphere gets no look in. When by chance it does get into the air, it instantly triggers our sense of smell. Human noses are so sensitive to it that HVO has reported that SO2 from the Leilani fissures had become undetectable by their instruments whilst they were still able to smell it.
Biology has a complex relation to sulfur. It is both essential to life (three amino acids contain sulfur) and deadly to it (it is potent antibiotic and insecticide). The strength of human hair comes from an S-S bond. Biological sulfur is mainly in the form of organosulfur compounds. These are food to anaerobic bacteria, and the waste products produce the smells which we find so offensive, signaling decay. Various animals use this to their advantage: it is the source of the repulsive smell of the skunk, and the socially disabling smell of garlic.
Magma invariably contains sulfur, as is already clear from the association between fumaroles and sulfur. But it is complex. Sulfur can dissolve into the magma either as S2- or as SO42-, depending on the oxygen content. The balance changes as the magma evolves. For instance, if magma degasses at high temperature, SO42- dissociates and SO2 forms, which stays in the melt. Mafic magmas (basaltic, from the mantle) can accommodate a higher level of dissolved sulfur than felsic magma (silicate, from the crust). Sulfur stays in the melt until it becomes saturated, and this happens later for basaltic magmas. But if basaltic magma incorporate some melted crust, it becomes saturated more quickly. The sulfur above the saturation limit is eventually expected to be outgassed into the atmosphere. In reality, things are more complicated. Sulfur concentrations in magma can be several thousand ppm. But what is emitted into the atmosphere can be ten to a hundred times more than what should be available in the magma. This sulfur problem suggests that a lot of sulfur congregates in a separate fluid inside the magma, rather than being incorporated in the melt. This fluid evaporates and provides the gas during the eruption.
Chemistry
Volcanoes mainly emit SO2, and some H2S. These are unpleasant, poisonous gasses. But over time, if they remain in the atmosphere they become sulfates, SO4 which forms areosols, very small solid particles. The chemical reactions that do this are not fully understood. Sulfates were part of the famous London fog of 1952, and are probably the reason that that fog caused such a high fatality rate (anything a volcano can do, humanity can do better). In the fog, sulfate may have formed from a reaction of SO2 with NO2, in the presence of high humidity:
SO2(g) + 2NO2(g)+2H2O(aq)→2H+(aq) + SO42− (aq) +2 HONO(g).
The role of the water droplets is because SO2 does not easily dissolve in water but SO4 does.
The haze enveloping many Chinese cities also contains sulfate, and there it is believed a second reaction contributes, involving NH3:
2NH3(g) + SO2(g) + 2NO2(g) + 2H2O(aq)→2NH4+(aq)+SO42−(aq) + 2HONO(g).
The SO2 can come from burning fossil fuels, while NH3 can come from traffic.
Volcanoes work in a different way. The eruption columns contain water (it is often rather wet), and gasses such as HCl quickly dissolve into the water and drop back to earth. But SO2 does not dissolve: it survives the eruption column and can reach high altitudes. A major eruption can move material from the volcano to the stratosphere in 10 minutes. That leaves little time for decent chemistry. The conversion from SO2 to SO4 by oxidization happens in the stratosphere and is a slow process. It takes 30 days before half the SO2 is converted. There are several reaction pathways possible and it is not well known which is the dominant one. One option uses H2O2, which is generally in short supply; another uses OH, and a third one ozone (O3). The reactions require water, and the dryness of the stratosphere is probably the reason why the sulfates take some time to form.
Once present, SO4 can nucleate to form microscopic aerosols (around 0.01 micrometer), which coagulate to become larger particles.
Even in the absence of volcanoes, there are always some aerosols present in the stratosphere. They come from carbonyl-sulfide (COS), which is present in the oceans. Sprays in the oceans brings it up to the air, where it survives long enough to penetrate the stratosphere. Up there, photochemistry begins the conversion to sulfate. But major volcanoes increase this continuous aerosol layer by orders of magnitudes.
Over time, the aerosols float down and enter the upper troposphere. This process can take 1-2 years. In the upper troposphere, the aerosols act as condensation nuclei, and trigger the formation of extensive cirrus clouds. This is very similar to the aircraft contrails which dominate the skies over the UK (it is easy to forget how much more common blue skies were in the pre-flight days. Over the past decades, there have been two occasions where the air was closed to commercial flights. Both times, the blueness of the UK sky was notable.
Sulfuric volcanoes
All volcanoes produce sulfur. Sulfur mines are in fact predominantly volcanic affairs; Europe used to get most of its sulfur from the slopes of Etna. The Bardarbunga eruption affected Iceland badly and raised SO2 levels even at times in Ireland. Effusive eruptions can be particularly bad because they don’t loft the gas as high: more stays at ground level.
The sulfur emissions from Kilauea are particularly well known. An overview of its emissions can be found at https://volcanoes.usgs.gov/observatories/hvo/hvo_volcano_watch.html?vwid=1379. To summarize, during the Pu’u’O’o eruption, the emissions averaged around 2000 tons of SO2 per day, although during the initial fountaining it as as high as 30,000 tons in a day. The outlook vent increased the emissions to 5000 tons, but at the expense of Pu’u’O’o where the emissions strongly declined. The lava clearly degassed at the summit before traveling down the rift. The Puna eruption was a sulfur disaster. Summit emissions went to 10,000 tons SO2 per day, but the Leilani rifts managed as much as 50,000 tons per day. After the eruption ended the emissions went down to less than 1000 tons per day, mostly from the summit. Before 1986, it was a few hundred tons. It shows how variable the emissions are, and how closely tied to the eruptions. The impact has been clear. Downwind from the lava channel, the vegetation has lost all it leaves. Upwind, the landscape has remained green.
Above we gave a number of 9 Tg of sulfur per year for all volcanoes. (The number for Hawaii given above is actually for SO2 rather than S: including the oxygen doubles the mass.) The average emissions of 2000 tons per day gives 0.36 Tg of sulfur per year. Kilauea in its normal state (Pu’u’O’o) is therefore not a major source of sulfur for the world. The Puna eruption is more significant: it produced roughly 1.5 Tg of sulfur, or one-sixth of the entire average volcanic produce per year. Still, it is very minor compared to the human production, bad for Hawaii but insignificant further afield.
What about the rest of the world? The image below shows the main volcanic sulphur emitters of the past 40 years. Kilauea is there, but is not the largest. The caption has a link to a movie showing when the eruptions happened.

From https://volcano.si.axismaps.io (the link shows a nice movie). Blue: earthquakes; orange: volcanoes; green: SO2. The symbols show events between 1960 and 2017; SO2 monitoring only started in 1978.
Going back further, the largest emitter over the past 300 years was Tambora, with an estimated 60 Tg of sulfur. Laki ranks a close second: it produced some 50 Tg. Other major events were Krakatoa (16 Tg), Pinatubo (8.5 Tg) and Santa Maria (6.5 Tg). (Remember that 1 Tg is equal to 1Mton: both units are used in the volcanic iterature.) These values are not particularly accurate, by the way. As a rough indication, a VEI5 explosions will produce 1 Tg of sulfur, and a VEI6 some 20Tg, but with large variations. It seems that the sulfur comes from a different part of the magma chamber, and therefore does not scale linearly with eruption magnitude.

Map of Io, made by the USGS and the British Astronomical Society. The active volcano Pele has a lava lake.
Vog
One of the many benefits of the end of the Puna and Pu’u’O’o eruptions is that Hawaii no longer suffers from ‘vog’. The word stands for ‘volcanic smog’ and refers to the mist of sulfur particles that hangs around, reduces visibility and irritates the throat. When the outlook vent opened in 2008, the vog around the crater went through the roof (figuratively speaking) and health concerns led to the closure of the crater rim drive (the explosion hurling rocks on to the parking area was also a factor, of course). The trade winds tended to blow the vog southwest, keeping the main populated areas clear but Pahala was in the line of the sulfurous haze. When the trade winds died down, as happens regularly, the vog would spread elsewhere and affect Hilo. An interesting tidbit: the trade winds only extend up to 2 or 3 kilometers. If the haze gets higher, it spreads the other way, towards America. Kilauea was not active enough to reach this far. But Mauna Loa is much more energetic and is also much higher. Its eruptions inject directly into the higher air flows.
The expression ‘vog’ first appeared in the mid 20th century. The effect has of course been known for far longer: it is not unique to Hawaii. In fact Hawaii gets off rather lightly. During the Bardarbunga eruptions, sulfur smells were noted in Ireland. And it can get much worse. Laki led to ‘vog’ across northwestern Europe. The word wasn’t known, of course: even ‘Smog’ (originally smoke fog, before it became a byword for L.A.’s chemical haze) was still in the future. Instead these events were called ‘dry fog’. They were not just minor health hazards. Laki’s sulfur-smelling dry fogs became killers. Tens of thousands of farm workers died in the UK and the Netherlands, from breathing the toxic particles. Laki was the Volkswagen diesel engine of its time, and a repeat of Laki would definitely break European air standard regulation.
“The summer of the year 1783 was an amazing and portentous one, and full of horrible phænomena; for besides the alarming meteors and tremendous thunder-storms that affrightened and distressed the different countries of this kingdom, the peculiar haze, or smokey fog, that prevailed for many weeks in this island, and in every part of Europe, and even beyond its limits, was a most extraordinary appearance, unlike anything known within the memory of man. By my journal, I find that I had noticed this strange occurrence from June 23 to July 20 inclusive, during which period the wind varied to every quarter without making any alteration in the air. The sun, at noon, looked as blank as a clouded moon …” (Gilbert White, 1788 “Letter LXV.“ The Natural History of Selborne )
This dry fog was at ground level and the sulfur directly affected people and plants alike. The aristæ of the barley, which was coming into ear, became brown and weathered at their extremi- ties, as did the leaves of the oats; the rye had the appearance of being mildewed; so that the farmers were alarmed for those crops . . . The Larch, Wey- mouth Pine, and hardy Scotch fir, had the tips of their leaves withered; . . . The leaves of some ashes very much sheltered in my garden suffered greatly . . . All these vegetables appeared exactly as if a fire had been lighted near them, that had shrivelled and discoloured their leaves (Cullum, 1786. Philosophical Transactions of the Royal Society Abridged volume 15, 604.)
Many people in the open air experienced an uncomfortable pressure, headaches and experienced a difficulty breathing exactly like that encountered when the air is full of burning sulphur, asthmatics suffered to an even greater degree. […] the fog caused a great extermination of insects, particularly amongst leaf aphids. (Brugmans 1787, A physical treatise on a sulphuric smog as observed on the 24th of July 1783 in the province of Groningen and neighbouring countries., Leyden).
Europe did not know what had hit it. People attributed it to a great meteor, or the earthquakes in Italy. It took three months before news from Iceland reached Europe and rumours began of an eruption of Hekla. The Laki fissure wasn’t discovered until ten years later, and the link between the dry fog of 1783 and Laki was only understood after Krakatoa, a century later.
Stratosphere
If the particles reach much higher, they can become stratospheric. A volcano manages to reach the stratosphere about once every other year. On average, a tenth of the volcanic sulfur emissions reach the stratosphere (1Tg per year), but it is concentrated in a few big explosive events which can increase the sulfur load of the statosphere to as high as 100 times the normal values. And if it is a large eruption, the sulfate aerosols can quickly spread around the Earth. This gives the milky, hazy skies known, for instance, from the Tambora eruption. Equatorial eruptions can spread the haze worldwide; eruptions at intermediate latitudes tend to stay within their hemisphere, and polar eruptions tend to stay above the polar front. Famous white skies occurred in 1257 AD, 538 AD, and 44 BC. But this only happens after massive explosive eruptions.

Sunrise on September 4th, 2008 over Hockley County, Texas, showing the colours of sulfur aerosols in the stratosphere from the Kasatochi volcanic eruption on August 7th (Aleutian Islands, Alaska). From http://www.mesonet.ttu.edu/cases/Ash_090408/Kasatochi.html
Stratospheric sulfur comes almost exclusively from subduction zone volcanoes. Spreading centres do not contribute, because their eruptions tend not to be explosive. The basaltic eruptions from oceanic hot spot volcanoes, such as Hawaii, also tend to be effusive rather than stratospheric. Their impacts remain more localized.
Flood basalt eruptions
Flood basalts are the largest eruptions on Earth. They are basaltic, and cover huge areas under kilometers thick layers. They are obviously not single eruptions, but a series of fissure lava flows, each perhaps 50 meters deep. Famous examples are the Siberian traps, the Deccan traps and the Columbia flood basalt. They often coincide with a mass extinction; in the case of the Siberian traps, one that came close to sterilizing the world. Can that be due to sulfur poisoning?
The flood basalts are similar to Icelandic fires, and form effusive eruptions from long fissures. They are on a different scale though, where the fissures may be 100’s of kilometers long and the lava flows can reach over 500 kilometer. The uniformity of the flows suggests that they barely cooled over that distance. There are two main models to explain this. In the first, the lava flows so fast that it has no time to cool. The second model suggests a much slower process where the lava flows underneath a solid crust, like the Kapoho flow field in its later stages. As an example, the Roza member of the Columbia basalt covers 40,000 km2 and has a volume of 1300 km3. The first model requires eruption rates of 1 km3 per day per kilometer of fissure, with a duration of weeks. In the second model, the eruption is much slower and lasted 10 years. That makes quite a difference, although it should be noted that in the real world, eruptions slow down as they age and the pressure behind them reduces.

Columbia flood basalt, the Roza flow. http://volcano.oregonstate.edu/book/export/html/486
The Roza basalt is estimated to have emitted 9,000 Mt of SO2, which may have been emitted over weeks or over 10 years. Compare this to the Puna eruption with its 3 Mt over 2 months: even if the Roza eruption lasted 10 years, that would still have outperformed Puna during its eruption by a factor of 60 – and would have continued 50 times longer. Tambora ejected some 60 Mt of SO2, and did so within 24 hours. Puna pales in comparison, at a paltry 0.05 Mt/day at its peak. On a daily basis, that is 25 times more than Roza (assuming the slow model) but over a year, Roza outperformed Tambora 15-fold – and it continued for another 10 years. Another comparison is Laki, which emitted some 100 Mt of SO2. If the majority of this was emitted within one year, the Roza eruption would have been nine times worse over that year, and it lasted ten times as long. Laki killed tens of thousands of people in northwestern Europe; Roza would have been much worse. We have seen nothing like it.
But how badly would this have affected the world? Effusive eruptions do not normally reach the stratosphere. The fissure eruptions would have send sulfur 5-10 kilometers up, but not much higher. The amount of cirrus would have exploded, probably over the entire northern hemisphere. This is not as effective in cooling the Earth as it would be in the stratosphere, because the intercepted heat still warms the air, but it would affect the air circulation and reduce thunder storm activity. Could the air itself become lethal? At concentrations of 10 ppm (10,000 times the level that our noses can detect), death can happen within hours. Lethal levels for prolonged exposure are not readily available, but can be assumed to be lower: 1 ppm (2mg/m3) is disabling even for short durations. Assuming that Roza erupted at a constant rate over 10 years, and that the SO2 lasted a month in the lower atmosphere, there would be 90 Tg in the air at any one time. If distributed in the lower kilometer, this would give a lethal level as far away as 5000 km. Ouch. A better calculation is obtained by assuming that the SO2 is spread out uniformly at 10 km/hr. Assuming a constant eruption, this gives a concentration of 80 ppm at 10 km, and a disabling radius of 800 km. (This also gives a limit for Leilani of 10 mile, which is reasonably close to reality. The numbers reduce if much of the SO2 is lofted into the upper atmosphere.)
Therefore, a flood basalt is deadly within 500 km, and ecologically devastating over as much as 1000’s of kilometers, but at this rate it doesn’t wipe out the world. Things are worse in the fast model where eruption rates are so much higher, but even there the effect is limited by how far the SO2 can travel before it drops out of the air. The winds on Earth are mainly east-west and so regions at the same latitude will be worst affected, and are areas far north or south much less so. The equator would act as an additional barrier, unless the eruption is in the tropical regions. A repeat of the Colombia flood basalt would wipe out much of North America, but leave most of the rest of the world alone.
It seems plausible that although the SO2 emissions from flood basalt eruptions were very damaging, they were insufficient to cause worldwide extinctions. Adding in CO2 may do just that, though, as it lasts much longer in the air and can cause severe overheating. The devastating extinction at the time of the Siberian traps were indeed caused by heat.
Final words
Sulfur is part of every volcanic eruption, and can turn an eruption into a killer. The gas spreads out further than the lava itself and affects many more people. Worldwide, our emissions far exceed those of volcanoes. Locally, volcanoes are important, as shown by the vogs of Hawaii, but worldwide, we now have other sulfur sources to worry about. Explosive eruptions can seed the stratosphere with sulfate aerosols and for a few years badly affect the climate. And looking back, the major flood basalt eruptions can have poisoned a significant part of the continent they occurred on. But the major extinction events attributed to flood basalts can not have been caused by just sulfur. CO2 is the more likely culprit. Still, volcanoes badly affect the air we breath. They smell.
Albert, August 2018
Which is why I endured the nips from his anger. I think he cued in on the smoky aroma. I smoke my own Chipotle, and it probably reminded him of some brisket trimmings I’ve made.
I’m finding it really interesting to follow the increase in activity in Öræfajökull. We are a week into september, and there have already been more quakes (M>1.2) than the entire month of July of last year, which was the first one to turn “yellow”. I don’t harbor any fear that it will go kablooie next weekend or anything, just saying it’s interesting to follow the increasing activity
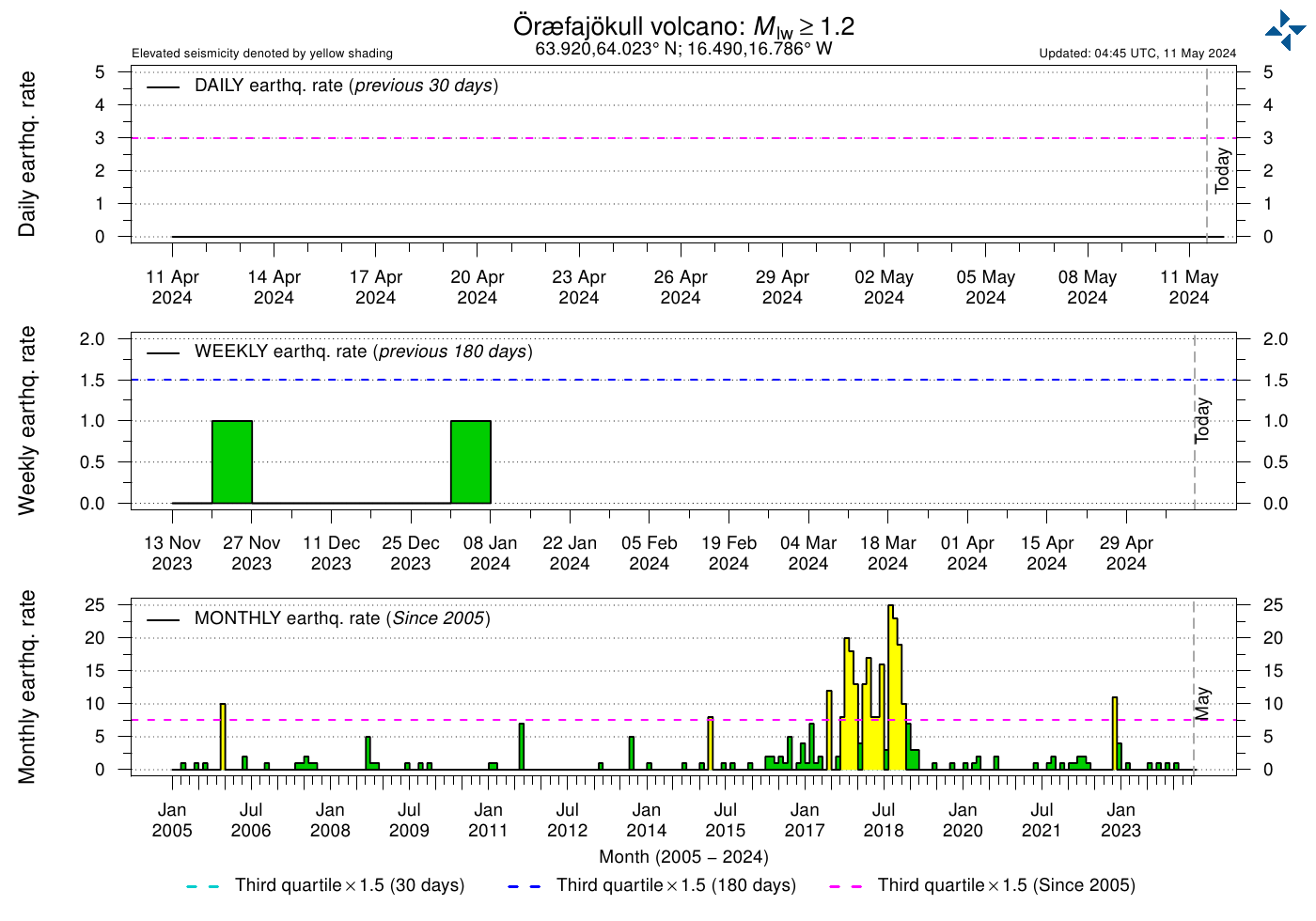
And now a new, 4 x Star swarm at Kolbeinsey north of Iceland, that continues into Grimsey and Tjörnes fracture zone. More to follow???
And last week more stars further north from Kolbeinsey..
Now 9 stars around Kobeinsey
i know this is a volcano place but i found this worth sharing to intelligent people…. also shared it to the air around me 😉 in a “Wow’…… https://www3.nhk.or.jp/nhkworld/nhknewsline/backstories/expertsmegaquake/
New(ish) post is up. Back to Iceland, with an expanded repost of the Surtsey eruption
https://www.volcanocafe.org/surtsey-the-birth-of-the-modern-world/