In spite of being posted on VC, the word ‘volcano’ does not appear in this post. Or at least, it wouldn’t have had I not written this preface. Which is why I wrote it, since we can not have a VC post which lacks the word ‘volcano’. (Of course now that I have mentioned ‘volcano’ twice (sorry, thrice), this apologetic preface is no longer needed!) This post is on popular request, as part III of the 2-part series on plumes and hot spots. I was asked to dig deeper. Here we go: to explore the core.

To the Centre of the Earth! There is actually quite a lot wrong with this book.
Imagine digging through a glacier of ice to a sea below. We are thinking about doing just this on Europa, the moon of Jupiter: it is covered by a 50-km thick layer of ice, with a liquid water ocean below. The only way to get into the ocean is by digging. In fact, we now know that many of the larger moons in the outer solar system contain such buried oceans, worlds of water we knew nothing about. There is a suggestion that even Pluto may have one, although that is rather speculative. And the Earth too has a hidden ocean underneath its coat of rock, at a depth of 2890±3 km below the surface, or 3485 km from the centre of the Earth.
But what a strange ocean it is. The dense rock of the mantle, denser then the densest basalt on the surface, floats on it. The ocean is hot. While the bottom of the mantle has a temperature of perhaps 2200 °C, the liquid below is more than 3500 °C – perhaps even as high as 4200 °C. There is a jump in temperature of at least 1300 °C! What on Earth is this liquid?
As befitting the surface of an ocean, the core-mantle boundary is flat, varying by less than 10 km in height over its surface. Most of that variation stems from a bulge at the equator, caused by the rotation of the Earth. We see the same effect on the surface of the Earth where the equator is further from the centre of the Earth than the poles are. (One of those funny sentences where the meaning changes if you write one word with a capital.) The local topography varies by no more than 500 meters from place to place. The top of the core coincides with the bottom of the Dʺ layer – the top of the Dʺ layer is much less flat. The Dʺ layer is one of the mysteries of the Earth. One suggestion is that is partly melted by the excessive heat from below, and perhaps even chemically altered by the interaction. Another suggestion (see below) is that it comes from a violent interaction. But interaction with what?
The outer core
The earliest speculation about the core of the Earth dates from long before its discovery. The story begins in 1798 when the first calculation of the density of the Earth were done. Remember that density equals mass divided by volume. We know the volume of the Earth very accurately (well – that excludes the flat-earthers who haven’t solved that basic problem yet). But how do we know the mass? Isaac Newton showed us how to do it: we can use the orbit of the Moon to calculate our mass. That is not at easy as it sounds. Newton’s law of gravity (derived from Kepler’s laws) only gives the product GM, where M is the mass and G is the ‘gravitational constant’ which you still need to measure. You do that by measuring the force of gravity between two large metal balls. It is a very difficult experiment because gravity is such a weak force. You may feel all kinds of forces between you and the person sitting next to you, attraction, repulsion, electric shock from their jumper, magnetic force from their iron piercings, but gravity is not one of them. Even if that person is of above average weight, gravitational attraction will still not move you. You would need to have the total human population of the world at 2 meter from you before the attraction you felt would reach even 1% of the Earth’s gravitational force. No, neither emotional attraction (or repulsion) nor peer pressure need to give any consideration to the gravity of the situation.
Still, people did manage to measure at least an approximate value for G. As early as 1798, this gave a mass of the Earth accurate to a few per cent (in hindsight). And as the volume of a spherical Earth is easy to calculate, this gave them the density of the Earth. This turned out to be 5.5 times that of water, or 5500 kg/m3 if you prefer. And that was a problem.
The densest rocks that we see at the surface are mid-oceanic basalts. They have a density approaching 3000 kg/m3. The measurement showed that the Earth overall was almost twice as dense. This could only be if a large part of the Earth consisted of something much denser than rock. Something that was very different from what we found near the surface.
The idea that the Earth had an iron centre developed at the end of the 19th century. It was based on the fact that some meteorites consisted of iron rather than rock – so perhaps the Earth was like an iron-nickel meteorite with a thick coating of rock. However, our actual knowledge really started with the work of Richard Oldham in 1906. In his paper, he writes “Many theories of the earth have been propounded at different times: the central substance of the earth has been supposed to be fiery, fluid, solid, and gaseous in turn, till geologists have turned in despair from the subject, and become inclined to confine their attention to the outermost crust of the earth, leaving its centre as a playground for mathematicians” before stating that “the subject is, at least partly, removed from the realm of speculation into that of knowledge by the instrument of research which the modern seismograph has placed in our hands”
He was in fact a seismologist, and had previously shown that earthquakes produce three different types of waves, now known as P, S and surface waves. In the 1906 paper, he begins with stating that P and S waves only becomes separated at around 1000 km from an earthquake, and that this ‘sorting out’ (as he calls it) can only happen in a more uniform material than found near the surface. In a spherical Earth, the line of travel to more distant locations goes deeper into the Earth. (I have no idea how flat-earthers accommodate this). At a distance of 1000 km, the waves arriving there had traveled through a deep region which is much better behaved than the crust. From his data, he found that the crust could be ‘no more than a few score of miles thick’, and that below it lies a different structure (now known as the mantle). (Oldham even shows that the crust is less thick around Japan than around Europe, so in a way he discovered the difference between oceanic crust and continental crust.) In the 1906 paper, he analyzes arrival times at seismic stations across the world, for a number of large earthquakes. They include the 1899 Alaska earthquake, and a large quake near the Moluccas islands in Indonesia in 1899 for which he used data from the mining engineer Rogier Verbeek – well known as the Krakatoa eruption expert, the only person who had had an inkling of the danger of that mountain.
Oldham’s result is shown above. Two curves are shown for arrival time versus distance, where the distance is measured in degrees (where 180 degrees is the opposite point on Earth). The bottom curve is for the faster waves (now known as P) and the upper one for the slower waves (or S). Up to a distance of 120 degrees, the change in arrival time is smooth. The more distant waves have traveled deeper into the Earth, and from the arrival times Oldham deduced that the waves traveled faster at greater depth but did so smoothly – there was no indication for a change in structure or composition, just changing pressure and temperature. But at 120 degrees, there is a sudden change. The waves slow down dramatically and there are distances where the waves are not seen at all, as in a shadow. From this, Oldham derived that the Earth must have a core with a very different structure to the outer region. His value for the radius of this core was approximately correct. What the core consisted of, he did not speculate on. Some claim that he discovered that the core was liquid, but that is not in the paper. In fact, that fact was not established for another 20 years. The propagation of the waves is illustrated in the first panel below, where the lines show the wave trajectories (note that in reality they are curved, something ignored here), the inner red circle is the core and the brown arc is the shadow zone.

The pattern with an inner core where reflecting waves can reach the shadow zone.
The next major step in the unraveling of the core came from Inge Lehman, in 1936. She was head of the department of seismology at a Danish institute. Lehman used seismic waves from a major earthquake in New Zealand in 1929, detected in her home country of Denmark. They shouldn’t have been there: from New Zealand, Denmark is in the shadow zone which the waves cannot reach. She showed that this could be explained by waves reflecting inside the core, but that required a surface to reflect from. In other words, there was another transition zone, and there was something else inside the core. It is depicted in the second pane, where the black lines show the reflections. Her model was so convincing that it was adopted within a few years. Now the Earth had both an inner and an outer core.
Lehmann was a remarkable scientist, both famous and under-appreciated, in a world where science was largely a male profession and many of them were strongly protective of their own gender. She never got the professorship she fully deserved, although later on she did receive several medals. Lehmann reportedly once said, “You should know how many incompetent men I had to compete with — in vain.”

The Maruia Falls in New Zealand (The South Island), formed by the 1929 earthquake which led to the discovery of the Earth’s inner core. It is a monument to science and deserves to be a protected UNESCO site – protected against future earthquakes! But even though it is one of the ‘must see’ New Zealand waterfalls, you will not find its role in the discovery of the Earth’s inner core mentioned anywhere. To the viewers, it is just falling water.
It was a small step to combine the lines of evidence for a high density component of the Earth and those for the existence of a core. This became the model of an iron core embedded in a mantle of rock.
Not all of the discoveries were made with seismographs. One of the most important findings came from the tides. We all know how tides affect the oceans – a source of endless play for children. But the Earth itself also has tides. The rocks underneath our feet move up and down by centimeters due to the tidal forces. How much the Earth responds to the tidal force depends on how strong the planet is. In particular, it depends on the shear modulus (see part I), its resistance to deformation. Already in the 19th century, Lord Kelvin used the earth tides to show that the strength of the planet (or its rigidity) is greater than that of glass but less than that of steel. By the 1920’s seismology had shown (from the velocity of the S waves) that the mantle had a much higher rigidity than this. The only way that the two results could be made to agree was if the core was very much less rigid. In fact, a fluid core was needed. This became the basis for the discovery that the core of the Earth was liquid. Once the inner core was discovered, it quickly became clear that this part was solid. And that is the story of how we came to know about the ocean under our feet, caught between the solid surfaces of the inner core and the mantle.
So the Earth in fact has two cores, just as the mantle has two hearts (part II). The outer core has a radius of 3485±3 km (compared to 6371 km for the planet) and the inner core has a radius of 1220 km. (The shapes are in fact ellipsoids, so the radii vary from pole to equator. The quoted radii are for spheres with the same volume as the real thing.) The temperature ranges from 3700±500°C at the core-mantle boundary, to 5000±400°C at the top of the inner core and 5500±400°C at the centre of the inner core. The outer core is liquid with a fairly low viscosity, perhaps similar to a low viscosity lava. The inner core, as already mentioned, is solid, sitting at the centre like an ancient Stone of Scone. But also, and this is much more like a black hole, it is growing and will eventually devour the outer core.
Composition
The original idea of a pure iron core doesn’t quite work. If you make the core a ball of pure iron, the Earth becomes a little too heavy. The core is about 10% less weighty than iron, so there needs to be a fraction of a lighter element included.
By the way, the density is of course affected by the sheer weight of the world above, including the weight of humanity. Material deep down is under considerable pressure and this compresses it a bit. The compression makes the density higher. But that compression is easily corrected for. The ‘uncompressed’ density of the Earth is very close to 4000 kg/m3, compared to the 5500 kg/m3 in reality. In case you are interested, the uncompressed density of Venus is very similar (perhaps a fraction lower), that of Mercury is higher at 5000 kg/m3 and that of Mars is lower 3700 kg/m3. Mercury appears to have a large core compared to the size of the planet, and Mars a smaller (or lighter) one. (Mars’ core was recently reported to be 1850 km in radius, from InSight data, making it around 55% of the radius of the planet. This is larger than had been expected. The ratio is now the same as that for Earth (also 55%). So Mars actually has the same size core (relative to the size of the planet) as Earth. However, Mars’ core may have a lower density than ours. Not all cores are the same – but they have a lot of similarity.
Based on what we find in iron meteorites (I have a small fragment of one at home but wouldn’t dare to use it for a destructive analysis!), the Earth core would be expected to contain iron mixed with between 5% and 20% of nickel. This is plausible but it is impossible to prove, as iron and nickel cannot be distinguished with seismology.

The Canyon Diablo Meteorite (http://deltaplanetarium.blogspot.com/2018/01/identifying-meteorites.html)
However, this iron/nickel combination is too dense by about 5%-10%, at least for the outer core. The density of the outer core ranges from 10,000 kg/m3 at the outer edge to 12,000 kg/m3 at the bottom (the increase with depth is caused by the higher pressure). Molten iron at these pressures and temperatures should have 11,000 to 13,100 kg/m3. This leaves a 10% deficit. The precise values do depend on the assumed temperature and some recent studies find a lower deficit of around 5%. But in either case, we need some lighter element mixed in – making it more like steel than iron.
Which element(s) is not known with certainty. Sulfur is plausible. It is depleted in the mantle, and the missing fraction could easily have gone into the core. Meteorites often contain iron-sulfide. But silicon has also been proposed, and even oxygen is possible if you can imagine a rusted core. Models can reproduce the properties with a range of compositions, with silicon from a few per cent to as high as 17%, oxygen at a few percent, and sulfur at 5 to 15%. These elements are to some degree mutually exclusive. The precise content remains a matter of speculation, as Richard Oldham would have pointed out.
The inner core also has a density deficit compared to iron-nickel, but it is less than that of the outer core. It is solid, and the solidification will have removed elements with lower melting temperature, which in general are the lighter elements. The evicted elements have to go somewhere, and over time this may have increased the fraction of light elements in the outer core. For the inner core, the best guess at the moment is 5% nickel and 3-7% of light elements which may again be sulfur or silicon. It is even possible that there is a substantial amount of carbon in the inner core: this has recently been suggested, but seems speculative as during the formation of a planet, carbon tends to end up near the surface. Hydrogen has also been suggested. The only certainty is that when we finally get our hands on a bit of inner core, there will be surprises.
The outer core is liquid and is expected to be well mixed. But the inner core may have different compositions at different depths. Perhaps we have a plurality of inner cores.
Magnetic field
The core of the Earth affects more than just seismology. It has given us the Earth’s magnetic field, providing a sense of direction, helping birds to migrate, protecting the atmosphere against the solar wind and protecting our electricity cables against coronal mass ejections.
The Earth’s magnetic field is generated in the outer core. Even though the core is iron, it cannot have a permanent magnetic field because it is too hot: an iron magnet looses its magnetism above a temperature of around 700 C, far below that of the core. Instead we have a dynamo: a circular current which generates a magnetic field inside the current loop. This magnetic field captures the intrinsic magnetism of the iron, and aligns it with itself. It also forces any charged particles (electrons) to move in a loop around the field lines, and these particles cause a current which strengthens the magnetic field, etc. Once the field is there, it continuously re-generates itself in this way.
The dynamo is started off by a combination of the rotation of the Earth and by convection inside the core. Imagine a blob of liquid iron moving around in the core. It is taking part in the daily rotation, and therefore moving at some speed, going around the world in one day. But as it changes location, the speed it needs for this changes. If it moves closer to the equator, it finds itself going too slow and therefore lagging behind. If it goes closer to the poles, it goes too fast. The same effect happens on the surface of the Earth. In fact this is what causes the air to move around in circles around low pressure or high pressure systems. It is called the Coriolis force, but it is all about keeping up with the locals. The same effect also happens when a blob rises or descends, and again finds itself with the wrong speed for the rotation of the Earth at its new location. In a highly viscous material, friction would immediately fix the problem but the liquid core has low viscosity.
The upshot is that the moving blob will be going in a large circle. And if it carries a charge, then there will be a circular current and therefore a magnetic field. That magnetic field will strengthen itself by grabbing the free electrons and forcing them into action, and the dynamo is in action. Details of the precise mechanisms are still being discussed: this is the general picture, but the interaction between the three processes (convection, currents and induced ferro-magnetism) remains an area of study.
The direction of this field is not pre-determined. The circles are shaped by the rotation of the Earth, and on average this will cause a polar field. But individual convective circles can deviate far from this direction. And the circles north and south of the equator will go around in opposite ways (just like low pressure systems on Earth) and produce opposite fields. So there is quite a chaotic situation in the core. As the dynamo takes hold, it begins to straighten out the field – but it can be many degrees off from the polar axis. And still has to decide whether to point north or south depending on which hemisphere wins out! Let battle commence – may the strongest field win.
The winner is not forever. The convective bubbles are still moving, sometimes strengthening and sometimes weakening but always distorting the field. The magnetic field may weaken enough that the battle between north and south is fought again. Most often, the existing field eventually wins out, but sometimes there is a different winner and there is a reversal of the Earth magnetic field. And suddenly all the birds which rely on magnetic sensors, find themselves at risk of migrating the wrong way. The book of Moby Dick tells how lighting reversed a compass so it pointed in the opposite direction, sending a whaler off course. Reversals are rare and may not happen for a million years or more. But they are a random inevitability. As far as we know, the Earth’s magnetic field has been present for at least 3 to 4 billion years, at more or less its modern strength. All this time, there will have been reversals.
But there have been some periods where there were no reversals for as long as 50 million years. How can that be? Perhaps these were times of reduced mantle convection, reducing the heat flow through the mantle which in turn could reduce the vigour of the core convection. But the precise opposite explanation has also been suggested! Another option is that the mantle convection at these times was stronger in the north or south, perhaps because of the location of the continents. It is speculation – we just don’t know. The core convection may be suppressed below the LLSVPs (remember them?) because they are hotter, or the LLSVPs may be hotter because of stronger upwelling in the core below them!
The magnetic field requires both a convecting liquid and a rotating planet. There is one more requirement: it needs an energy source. Nothing comes for free. Where does the energy come from? There are two energy sources which drive the Earth core’s convection. One is the heat from the inner core, and the other is buoyancy caused by the loss of heavy elements to the core below and light elements to the mantle above. At the top, magnesium from the liquid core may attach itself to the mantle, leaving the core liquid a little heavier and ready to sink. At the bottom, the heavy iron and nickel may freeze out on the inner core, leaving the liquid there a bit less dense and ready to rise. This process can drive convection, and models suggest this is the main source of energy.
And as a final point: because the convection itself carries the magnetic field around, we can actually trace the convective flows in the outer core from the changes in the magnetic field at the surface. In the plot below, green shows descending flows in the core, arrows show horizontal flows and yellow/brown shows upwelling. It is amazing how we can map movements so deep inside the Earth. And notice the velocity, reaching a staggering 15 km per year. Well, it is staggering compared to the mantle doing a mere 0.5 meter per year. That is the advantage of being a liquid. It is giving our snails a run for their money.

Credit: Jeremy Bloxham
The inner core
Inside the liquid outer core sits the solid inner core. It hasn’t always been there: the inner core has formed by slow solidification of the outer core. At the moment, the inner core grows by about 1 mm per year. At this rate, in 2 billion years from now the entire core will be solid and seismology will become a lot easier, to those of us not as competent as Inge Lehmann, but navigation will become harder for lack of a magnetic field.
There are suggestions that the core growth is not the same everywhere, and that it is higher around the equator and lower at the poles, and also that it is higher in the western hemisphere. The cause is speculation! A possibility is that the rotation of the Earth causes stronger convection at the equator, meaning faster heat transfer and therefore faster core growth.
It is part of a general asymmetry of the inner core, which has seismic waves traveling along the poles being faster by between 2 and 9 seconds compared to ones traveling along the equator. (It depends on where on the equator the delay is measured, and that depends on where the particular earthquake originated!) (The lack of a subduction zone near the poles presents a problem, since it means we rarely have a strong earthquake with a seismic wave traveling very close to the polar axis.) The explanation for the asymmetry is hotly debated. As it solidifies, the iron forms crystals which can be rather large – they may be a kilometer or more. The crystal structure is a bit more rigid in one direction, by a few per cent. If all the crystals in the core have become aligned, this could explain the observed asymmetry. In fact, the faster equatorial growth might do this: it requires a slow creep along the core of the solidified material to the poles (to maintain the spherical shape of the core) and this creep could cause the alignment.
Some measurements suggest that the inner few hundred kilometer of the inner core have an opposite alignment, where the waves travel faster along the equator. This has been interpreted as an ‘innermost core’ inside the inner core. It seems to be re-discovered by different groups every few years, ever since the original finding 20 years ago, but whether it constitutes a distinct core region remains disputed. There is no clear and sharp transition radius in the data.
There is also a change in seismic properties some 200 kilometers below the outer edge of the inner core, which may relate to a phase change of the iron crystals.
The inner core appears to rotate slightly faster than the rest of the Earth, although only minimally so. The first measurements gave an excess of 0.2 degrees per year: like a sluggish version of Phileas Fogg, the inner core is gaining one extra rotation every 1800 years. Newer measurements has reduced it to 0.07 degrees per year. The faster rotation can come from the slight contraction during solidification. If a rotating object becomes smaller, it rotates faster.
A very recent paper claims that the inner core has changed from slower to faster rotation over the past few decades. That is a surprising result which needs confirmation. (A series of large earthquakes over the next few years would help!) . It is not impossible, and could arise from interaction between the convection in the outer core and the rotation of the inner core. A sinking blob attaching itself to the inner core needs to shed some of its rotation (angular momentum) and in doing so speeds up the rotation of the inner core. A rising blob would do the opposite and over time they average out. If this is correct, it would show how dynamic the zone between the inner and outer core is. We know from seismology that the top of the inner core attenuates seismic waves a bit more than expected. It is not a perfect, sharp boundary: the top of the inner core is a bit mushy and perhaps sticky. Maybe the La Brea tar pits are a good comparison.
Origin
The world underneath the mantle is a very different one, almost like another planet hiding inside ours. Like the Tardis, the Earth seems bigger on the inside. How did this happen?
The formation of the core requires that the iron separated from the rock, as in an ancient smelter, That required a molten Earth, a magma ocean where the heavier iron could drop to the bottom. But the only time the entire Earth was molten was during its formation, when the continuing collisions kept the planet hot. The core as we know it must be from this very beginning, 4.57 billion years ago. It appears that the formation of the core was largely complete before the Earth was 30 million years old, perhaps even before the Earth had reached its full size.
Some elements are called ‘siderophile’: in chemistry, they side with iron. Others are ‘lithophile’ (barium, rubidium) and side with rock (uranium, lead). When the core formed, the siderophile elements would have gone into the core, and these elements would become depleted in the mantle. But in the oldest rocks we know on earth, these elements have the same proportion as modern material. The depletion of these elements did not increase over that time, and this shows that the core stopped forming before these oldest rocks formed, more than 4 billion years ago.
Very early in its life, the Earth was hit by a Mars-sized body which we have optimistically called Theia. (In mythology, Theia is the daughter of Earth (Gaia) who gave us gems, but in the real world she nearly killed us and the debris of the collision formed the Moon – not quite the same thing.) Apart from the Moon, Theia must also has left remnants in the Earth. One recent paper suggested that the LLSVPs are these remnants – that is very unlikely. But there may be a signature in the core. The Dʺ layer and the upper few kilometers of the outer core are the most likely: the suggestion is that shock waves from the collision mixed the top of the outer core (at that time, there was no inner core) and the bottom of the mantle. The Theia impact appears to have happened around 40 million yers after the Earth began to form, at a time when the core of the Earth had already fully formed. The idea that the core is itself Theia’s remnant can be ruled out. The fact that the core of Mars and the Earth are so similar in relative size suggest that core formation is a normal part of planet formation, and not related to particular events such as this. The huge impact had little or no effect on the size of the core. It also means that the Moon is younger than the Earth’s core.
How about the inner core? At the current growth rate of 1 mm/year, it would only be 1.2 billion years old. There are a variety of estimates, ranging from 2.5 billion years at the oldest and 0.5 billion years for the youngest. In any of these estimates, the inner core is the youngest component of the Earth. Our liquid core is disappearing. This should be a major topic for the next election: what is the government going to be do about this? For if the entire core solidifies, as may happen in another 2 billion years, we would lose out magnetic field and that would be a major environmental disaster.
I propose an action group, called IronicAction, with slogans ‘Save Our Core (SOC); Cease The Freeze; Keep The Iron Curtain (KIC); Stop Iron Change (SIC)’. A Hollywood movie will be made about remelting the inner core, called Extreme Ironing. Scientists demand to limit core growth to no more than 2 meters, to avoid catastrophic change. The Navy spends 10 billion dollar finding new ways to sink its ships. Politicians will talk, decide, and inact (politics being the only activity where inaction is a verb). And Elon Musk develops plans to use the core as a giant battery. But that is a story for another, distant day.
Albert, June 2022
This post is largely based on the book by Ken Condie, The Earth as an evolving planetary system (4th edition), 2022, Chapter 5

‘The core’. There is an awful lot wrong with this movie.
Thank you albert.. excellent article
At 3800 to 5000 C depending on depth the outer core probaly have a very low viscosity similar to water I guess .. Iron have No sillica chain polymerisation at all as a liquid .. so Will have a very low viscosity. There is probaly gigantic hurricanes of Liquid Iron at the outer core spinned up by Earths rotation and combined with Earths spinn.
In 2 billion years the Sun will be 20% brigther so Earth Will be dead long before the core freeze up.
I do wonder about the core temperature of Massive Super Earths.. must be very high: Kepler 277b probaly sits at tens of thousands of degrees C ! And Maybe one huge liquid ball at the center .. probaly have a magnetosphere that is very powerful as well
The mass of this particular planet is quite uncertain. But we can make a guess from the radius. It is about 2.5 times the radius of Earth, and if we assume a similar temperature gradient (which would not be unexpected, but if the mantle would be liquid the temperature gradient will be less), then we get a core temperature of between 10,000 and 15,000 K. But it is guessing.
So a liquid mantle carries away heat more efficently right .. as it can convect very quickly
Still Kepler 277b is ..perhaps 70 / 80 Earth masses! I think We are talking about tens of thousands of degrees C in the center .. Earth itself gets to 5500 – 6000 C in the center with only one .. Earth mass
to bound,
look at the convection video in the article. Hot material is being dragged along by the flow above it and then dragged up at the sides only to fall (denser) in the centre. This downwelling is thus exactly what the model predicts.
The mass is probably not 70 or 80 earth masses. The possible range is quite large and my guess is that the most likely mass will be around 40 earth masses. And do remember that the cooling rate scales with inverse radius, not mass. The radius of this planet is actually better known, at around 2.5 earth masses. So make the central temperature 2.5 times that of Earth. Of course the age of the planet is also important.
The planet is also close to the star, much closer than Mercury (both of them actually) and the huge mass would mean the gravity is a lot stronger. Impacts will have much more energy, so presumably the accretion and bombardment will have heated up the planet much more than happened on Earth.
Is this an important factor or is heat flow within the planet too efficient?
Good question. You would assume that most of the accretion is with objects on very similar orbits. In that case the distance to the star does not matter much. If objects come in on highly elongated orbits from the outer system, then the location matters but this will be only a minority of impacts. The impact energy scales with the radius of the planet, so if it is 3 times larger (27 times more massive) each impact brings in 3 times as much energy. Larger planets get rid of their original impact energy slower than smaller planets. The Earth has lost ours, and the internal heat now comes from other processes (radioactive decay, lqtent heat, contraction). Actually, radio activity from other, shorter-lived elements is very important in the first 10 million years or so of a planet and it can cause even small objects (say 10 km) to melt. It is not only impact energy.
Interesting, so gravity is really determined by the actual physical size of the object than how much mass is in that object, at least when dealing with normal matter that planets are made of.
I did look, at least on Wikipedia Kepler 277b is listed as 87 M at a median, with a range of about 40 M either way.
That information is derived from https://exoplanetarchive.ipac.caltech.edu/overview/Kepler-277%20b#planet_Kepler-277-b_collapsible
So it seems the planet is actually that big, its lowest is 47 M and at the upper end it is 127 M, which is slightly bigger than Saturn. The star is of unknown age but is similar to the sun only slightly brighter and more massive, it is probably older on account of it being larger in radius, so formation heating in the planets is probably not relevant anymore.
I imagine both of these are the sort of thing that gas giants form from. Presumably the process that forms Hot Jupiters occurred here except maybe the star formed quickly so the planet was not able to accrete any of the nebula, and remained as a supermassive solid object.
The better value is the one from Hadden et al, which is roughly 80(+70-50) earth masses. (It was determined from more data than available for the measurement you quote, from Xie et al). Either way, it is a very uncertain value. Combining the mass range with the probability distribution for planet masses points at the lower at of the range as more likely. The radius is much better known and points at ~ 25 earth masses if our density, or perhaps 10- 15 earth masses if Neptune like (i.e. an ice giant). That kind of planet is not uncommon but lies a bit outside the uncertainties. Not excluded though.
It gives the density as about the same as platinum, 20 g/cm3. There are planets more dense than that, and some dim red dwarfs are even much higher still, Proxima and Trappist 1 are both 150 g/cm3 density, fitting over 100 Jupiters into a space that is the physical dimensions of 1 Jupiter… 🙂
Very different mass range. What you quote is for brown dwarfs, with masses approaching those of stars. Here we have an object that is perhaps 10% of Jupiter. Those tend to have densities similar to Jupiter, or for that matter of Earth.
Crystals are forming on its surface, so it’s probably fuzzy, a bit like an inside-out geode. That would act like a sound baffle at the side of a highway, especially for a wave with a grazing trajectory rather than going right through.
There is no strong correlation between the LLSVPs and the convection pattern in the outer core. The downwelling under the Pacific is not underneath the bulk of the LLSVP (which is further west) and the African one is near (but not at) both downwelling and upwelling. But do remember that the outer core changes fast, and that the LLSVPs are ancient. It is not clear that we should be looking at the current core pattern, or one from a few hundred million years ago.
What does the CMB do at LLSVPs? Dip, rise, or remain more-or-less level?
https://youtu.be/0ygdwSVsj68
Kilauea update, these guys are pretty great for information, it is where I get a lot of my knowledge from 🙂
Basically the lava lake we have now is an open conduit that is probably going to be there for decades, and that the rate of filling appears to be (slowly) accelerating. The rate of filling now is also very high, higher than Pu’u O’o but it can still get much higher, in the early 19th century it was filling twice as fast as it is now. Also lots more stuff too but not as volcano-related;
So if anyone wants to go see lava, this is the place, coming from a primary source 🙂
Its basicaly a Giant shield vent thats trapped in the caldera 🙂 the lava is getting hotter and hotter now it seems and the magma supply seems infinite
Probaly is as fluid as Nyiragongo according to the fluidal behaviour when rim falls into these lava lakes. The overlook lava lake behaved like water when pieces of the rim fell into that lava lake.
I dont think the lava is hotter, at least it isnt really hotter than any other point in ain at least the past year. The first lava in 2020 was compositionally the same as the 2018 lake but a slight bit cooled, but I really doubt it has stayed that way or even that it lastedmore than a few hours before the typical hotter stuff erupted, the samples were fountain fallout from high fountains which lasted only a few minutes in both the recent eruptions (fountain to 200 meters in September, but it lasted only one frame on the webcam).
The thing that caught my attention on the live is their graph showing the volume plotted over time, and how it is not only staying very constant but might actually be accelerating, the supply rate getting ever higher. 4.1 m3/s is equivalent to 350,000 m3/day and 0.13 km3/year, which is already more than the long term average for Pu’u O’o, and the GPS is still showing extension of the caldera. Unless there is a flank eruption I will be very surprised if any of the caldera floor still exists in 2030. If a rift episode does happen I hope it activates the southwest rift,
A piece of the wall actually fell in when I saw it, but it was not so impressive as the recent video, the lake was less active. I also was taking a picture so missed it, and the picture blurred 🙁
The filling up rate of the caldera in the early 19th century is not well known, I did my best calculations and found the eruption rate in 1823-1840 to be roughly the same as during Pu’u’o’o. The numbers in “Two hundred years of magma transport and storage at Kilauea”, are highly exaggerated for that particular period, I suspect they may have taken some estimates on the caldera dimensions made by 19th century visitors too seriously.
Regarding the situation now, the amount of inflation+effusion at the summit probably well exceeds the supply rate during Pu’u’o’o, however this is not unexpected because the Middle East Rift Zone storage complex has been deflating ever since the eruption started, in other words the Middle East Rift Zone is contributing magma to the summit which adds to the supply from depth. We are getting the magma that was supplied to Kilauea in 2018-2020 erupted, this magma had mostly went into the MERZ and is now going back towards the summit because of the low pressure there caused by the eruption onto low elevations. If it wasn’t for the MERZ storage Kilauea would have erupted sooner after 2018 I believe, because pressure would have built up faster in the summit rather than flowing downrift.
Probably not a good proxy for the actual shape of the ERZ storage, but a dike that goes from Mauna Ulu to Heiheiahulu, 23 km x 3 km and is 20 cm wide (how far the ERZ dilated before the eruption of 2020) it has a volume of about 0.13 km3. The summit in that same time dilated by 90 cm, it is though a circular structure so a dike would not work here, I dont know how to model it.
There was also some 80 cm of dilation during the big quake in 2018, going to about half that at either end of the active slide. That would be about 0.4 km3 compared to the above calculation. But then most or all of that was deeper, at the basal fault, so not sure if it woudl have done anything above the magma system at all. But then the widening of the rift in 1975 is believed to be what allowed Pu’u O’o to happen, through both rapid refilling from the summit as well as probably some amount of direct fill from the deep rift, the same thing presumably was happening before the eruption of 2020 and will occur again as soon as the two systems reach equilibrium.
Until we get the new USGS data on Pahala, and it says otherwise, I will continue to hold it as a warning of something big to come. Volcanoes vary in deep supply rate, sometimes by orders of magnitude. Kilauea has an average supply of about 0.1 km3, probably a bit more but close enough. It has also been much less than that, 0.02 about 100 years ago. But then we have not seen it go the other direction, perhaps on occasion it goes much higher than the base rate. Going the other way gives a rate of 1 km3 a year. Would only be very temporary, maybe for a few years, but that is enough to change things up a bit…
What would the early signs look like? I imagine a raging cluster of quakes active for years near the base of the crust and pointing in its general direction, might be quite a good hint.
Of course I think USGS is going to say something technically correct but rather unbelievable, like that the swarm is somehow not related to volcanism at all because it doesnt fit with their model.
The East Rift Zone storage consists mainly of two large sill complexes, so yes, a dike would not be a very good model. Together with the summit storage they make three sill complexes that exist on Kilauea. This is an interferogram from shortly after the 2018 eruption ended, in this time the summit chamber was still deflating, however the two ERZ sill complexes had rapidly started to inflate, visible as the two red balls. One is roughly under Pu’u’o’o, the other under Heiheiahulu, although they can get a little thrown off in interferograms by the look angle. The Heiheiahulu one is rarely visible in interferograms because its deformation is very weak, only when a new sill grows, in this case however, immediately after the 2018 eruption it inflated very fast making it noticeable. The Pu’u’o’o one instead has very strong deformation often making a signal larger than the summit, and not too far behind in amplitude.
Here is a close-up look on the sill complex under Pu’u’o’o (MERZ). It is a sill complex for two reasons: it has a bullseye pattern of inflation typical of sills and magma chambers, and it doesn’t caldera collapse so its not a magma chamber (yet).
And here is the Heiheiahulu sill complex getting an intrusion in 2020:
I got the interferograms from here, though it is not loading for me right now:
http://insarmaps.miami.edu/
The way I presently understand Kilauea’s plumbing is the following: The East Rift Zone has three “sill jumps” corresponding to where lava travels through the sill three complexes shown in yellow, as well as three “rectilinear sections” shown in red. The green section is where lava travels through the summit sill complex making a very robust tube that shows as a continuous band of seismicity in relocated earthquake catalogues, the so called East Rift Zone Connector, and it is oblique to the dike intrusions. The red sections are characterized by discontinuous bursts of seismicity aligned with the dike intrusions of the ERZ. Black arrows show the direction of sill propagation. My understanding is that sills propagate downslope, something I’ve learned from Piton de la Fournaise, and note that the ERZ sill complexes are located just north of the rift axis so probably prefer to go northeastwards through the northern flank of the ERZ. Summit sills so south or southwest and that has been seen repeatedly, in 2006, 2015, and 2021.
Basically magma flows along the dike swarms, for example flows from Aloi to Napau following the dikes, then when it reaches Napau, the end of the dike swarm conduit, it builds up pressure and makes sill intrusions, these sill intrusions allow it to jump onto the next rectilinear section which corresponds to one with very little dike activity, only 1977, and where dikes dip southward so that the conduit is offset to the south from the fissures manifestion on the surface. Just before Heiheiahulu, is the end of that conduit section and makes sills again which allow to jump to the third section, that makes up the core of the southern fissure swarm of the LERZ (1955, 1960, 2018). The way I think the ERZ may have slowly drifted southward I think might be because of those detached dike swarm sections migrating uprift towards the summit once the start of the dike swarm sections positions in front of the summit they coalesce with the summit sill complex and thus grow southwards the connector.
So there is a reason for everything. If I haven’t THE Kilauea article yet is because I keep finding out stuff. I’ve already known about the relocation seismicity catalogue and the insarmaps Miami for more than 6 months and looked at all the data. But then, when I think I know everything I let some time pass, and when I start to forget what I thought I knew, I look at it again only to see that I was wrong somewhere, or had missed some detail, or some better interpretation.
And here is the summit sill complex in full display. The northern portion is inflating (red) and the southwestern portion is still deflating (blue) to refill the summit chamber and ERZ from the 2018 drainage. It is quite big, and particularly it is long. 11,000 years ago the sill complex probably merged into a chamber and collapsed into a 30 km long caldera, leaving behind the Kaoiki cliffs.
The map above was basically a combination of relocated earthquake data from Robin Matoza’s wonderful catalogue, and the interferograms from the University of Miami website. Here are the relocated earthquakes, showing the three alignments of shallow, 2-3 km deep, earthquakes that are parallel to the dike swarms:
Been searching for insar maps younger than 2018 since about that year, never found anything, it is fascinating to see.
I guess by stretching the definition a bit Kilauea is a rift zone with 3 central volcanoes of which one is a primary and is in controll of the other two. I think though there would be some degree of vertical feed in the ERZ, meaning magma feed that doesnt come through the ERZ connector. That would actually seem to be confirmed by the deformation with the ERZ inflating rapidly separate from the summit.
I had seen an insar of inflation southwest of Kilauea before, but the area was not so big. It looks like basically all of the deep rift going all the way to Pahala is directly connected to the summit chamber. It is strange the area doesnt erupt that much and when it does it is a dike from the summit, one would expect abundant eccentric eruptions in this area as happen down in the LERZ.
It also looks like when Kilauea is fully mature and at its maximum size, it could be something that makes eruptions of Ionian scale… Wouldnt go as far to compare it to a LIP but closer to that than to a typical volcano. Loihi will continue to grow, and Kilauea eventually will build southward, one day its summit will be far inland like Mauna Loa today, which will slow rifting in that area and promote the formation of a gigantic magma chamber. I imagine also the final form Kilauea could have a second summit where the middle ERZ is now.
Maybe something like this.
Yes, the area to the southwest of the summit with storage is pretty large, however its southwestern end has probably very little sill density because deformation is very subtle there. After the 2018 eruption the southwestern end of the sill complex kept deflating to supply the summit and ERZ so it showed up more clearly.
I no longer think it is related to Pahala though or to the deep rift, if such a thing really exists (starting to think anything carrying the word mush is not real). It is simply sills that follow the steepest lines of descent from the central-south portion of Kilauea’s caldera. We saw this process in action in 2015 and again in 2021. The red polygon is approximately the 2015 sill, and the green polygon is the 2021 sill. They start from the location of the down dropped block and follow the steepest lines of descent, in this sense they are much like lava flows underground. They are the underground equivalent of the Observatory flows above, The yellow area makes up the whole complex which deflated in 2018 and afterwards. The red lines are the Kaoiki Pali scarps which probably formed when the whole sill complex collapsed about 11,000 years ago, and with a caldera volume of ~20 km3.
There:
Although now that I think of it the Pu’u’o’o and Heiheiahulu sill complexes couldn’t be starting from the main conduit and follow steepest lines of descent, if they did they would from southeastwards. So I guess either one of two options, there may be other factors that affect sill propagation, or they are outside the conduit, more to the northeast of it. Perhaps they are where old dike swarms have been replaced by more southerly ones but magma can still access. Well, that’s something to think about.
Would agree for Pahala not exactly connecting to the obvious surface expression of the SWRZ. But looking at the way the swarm has progressed in the past 6 years it has moved closer to Kilauea, along the same trend. There are also generally speaking more deep quakes of similar depth to the Pahala swarm trending along the SWRZ and upper ERZ/summit of Kilauea, there does appear to be something.
Not sure really how to explain Pahala as anything other than magmatic. A fault slipping would not be so noisy, or so long. While at very different depths the Pahala swarm does seem to have a hard northern limit not too far from the southwest end of the sill complex. Perhaps that complex goes down alot deeper at a steep southwest dip to meet with Pahala. Perhaps it also has a southwards dip along the whole south flank, like a stack of books that is stepped over. How far east this might go, or doesnt go, would be nice to know. It seems unlikely though that all of the magma in the ERZ comes from the summit through the connector though, the rift would be deeper than 3 km, and magma would be encouraged to flow that way at all depths above the basal fault. The ERZ connector is probably giving most if the hydraulic pressure to drive the eruptions at the intensity we see.
Okay, it took me to see the JOKA and JCUZ GPS to see where the sill complexes are exactly located. They are not in the north flank of the ERZ, that is an illusion created by the satellites which look from the north and make the bulge on that side. Actually the sills are on the southern flank of the ERZ. Right now JCUZ is deflating towards a deformation source to its southwest. JOKA whenever it inflates it does so from a deformation source to its southeast. This matches with satellites that look from the south like this, although the current JCUZ source of deformation is not active on this one:
There are actually at least three sill complexes aligned with the seismic middle portion of the East Rift. They can all be seen deflating in interferograms from the start of 2018 eruption
: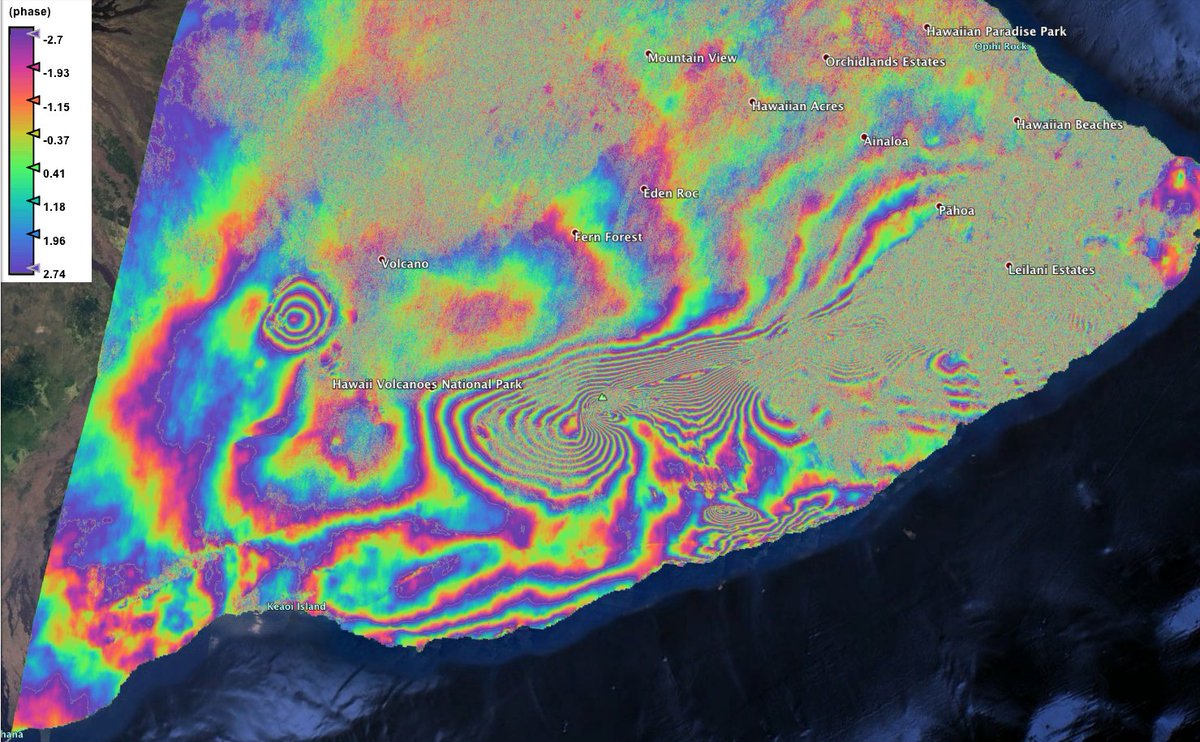
Pink balls are shallow earthquakes in the middle portion of the rift, yellow are the rough locations of the deformation bulges from the sills.
There is certainly something magmatic about the Pahala Swarm, but what? I was certainly wrong with the idea of a series of pulses arising that I gave on “Magma waves from the phantom rift”, so no longer do I know what to expect from Pahala. I’m starting to think that if it was really a surge of magma rising it should have arrived to Kilauea, long, long, ago, about early 2019. Kilauea’s system is open after all. Pahala could easily have been a deep sill or dike intrusion that has excited one of the many horizontal reverse faults under the south flank of the island, or who knows what exactly.
There is nothing wrong with being wrong. One has to be wrong a lot of times to be right some times after all. As long as you realize there was a better way to think about it. The idea was quite valid, and maybe still is, a lot of magmatic tremors under Pahala in early 2019, and then a lot of volcano tectonic earthquakes 10 km shallower in a region that is connected to Kilauea via the horizontal Mantle Fault Zone of earthquakes. There are multiple scientific articles out there claiming there is a connection from Pahala to Kilauea via the MFZ. But time has passed, and since 2019 it has given no sign this is progressing to anywhere beyond Pahala. So the idea is eroding away from my perspective.
When a surge actually happens I think the first thing we will see is Mauna Loa flaring up with deep LP earthquakes and then Kilauea will start doubling its CO2 emissions, and inflating faster. This is literally what happened in 2004-2006 which was probably a surge. It produced an extraordinary LP swarm 45 km under the summit of Mauna Loa, which there wasn’t any record of such a thing happening, and hasn’t repeated since, and shortly after Kilauea was making record high CO2 emissions (above what had been recorded up to then), which eventually peaked in series of intense sill intrusions to the southwest of the summit, and possibly increased effusion rates from Pu’u’o’o (according to an article from Michael Poland). Quite cool for a Kilauea fan, but not so much for anyone else.
I mean Kilauea has an open system, but it has always been an unproven assumption that it connects to Pahala already. One would really expect it to be more vertical, probably. Pahala may be something that began as its own thing, but cabt erupt at its location. Instead it is moving towards Kilauea through a fault zone but not an easy path. HVO has seen quakes here since records began but the swarm only seems to have become ‘magmatic’ in the past few years. I actually originally had an idea like this but based only on blind assumption, however it might be something to reconsider.
It might be something to look at how Holuhraun began. Bardarbunga has a robust plumbing system, and had been building for a long time. However what set off the eruption in the end was an intrusion going from the northeast back into the central volcano, overpressurizing it. To me Pahala is like this only the timescales and overall magnitude are much larger. Kilauea is not fed from Pahala, but there is magma at the latter which is trying (slowly but so far successfully) to move that way to escape. It really is just a question of when, my guess is the swarm will probably quite rapidly stop when it encounters a magma system that reaches the surface, followed by a rapid increase in activity on the surface.
It would probably help a lot if a working GPS existed over the swarm, even at that depth if magma is accumulating it would probably give a sign.
Intrusions themselves are largely aseismic, except for tremor and LP earthquakes, the actual volcano-tectonic earthquakes will happen on nearby faults. Faults right under a dike which accommodate the dike extension, or maybe caldera faults formed in collapses which are set in motion by an intrusion. Pahala and the Mantle Fault Zone are sub horizontal reverse faults which accommodate the southward slip of that side of the island, they have generated large earthquakes, there was an M 6 in that area last year. The Pahala Swarm and the MFZ are probably not intrusions and not magma pathways, but actually faults. Something has set in motion the fault section under Pahala, possibly some kind of intrusion or magma movement related to the tremors offshore Pahala that started in early 2019. But not knowing how the plumbing down there is structured it is hard to know its significance. It could be a Kilauea thing, but it could also be a Loihi thing, or maybe an intrusion limited only to Pahala.
I guess we will know what is going on under Pahala in not too long, even if the swarm itself is not magmatic there has to be force acting upon the area somehow, which is most likely magma. And given it flaring up not too long after a major eruption it would be probably more likely related to Kilauea than Loihi. It also could be Mauna Loa, but if Kilauea is a satellite of it then really anything that deep probably affects both of them.
One day I guess it will be possible to image the magma pathways. We can reasonably understand the surface levels but most surely it is not just a simple vertical pipe from 60+ km depth up to the magma chamber. Same in Iceland too, Grimsvotn and Bardarbunga are closer to each other than the thickness of the crust in that location, they may very well be connected at depth above the mantle like Hawaii could be.
What a fascinating article. Thank you! I’m really pleased to learn how the magnetic fields work, too. I was always a bit thick over those.
Excellent !!
Could you consider a follow-up explaining the red/yellow plot credited to J. Bloxham ?
The stuff in mid-Pacific seems associated with the immense volcanism there, but what is going on along India’s ‘skid-mark’ at 90ºE ??
Here is the 2021 Hawaii Insar Information. Sorry you will have to select the entire link before clicking it.
https://www.google.com/search?channel=cus5&sxsrf=ALiCzsZx2JW2xZR-gqSojOXfDw7cM_7oGg:1656275838598&source=univ&tbm=isch&q=insar+maps+from+Hawaii+younger+than+2021&client=firefox-b-1-d&fir=eEALnu63RbnZ0M%252C3l5U6gSBsPo_zM%252C_%253BaBwWSPvb9TpxtM%252CW1T72iDIFCaVYM%252C_%253BeH49BwpPJhVoIM%252CW1T72iDIFCaVYM%252C_%253B7FGAIJo9xnAxPM%252CDOi8K2WfELfE6M%252C_%253Bt8DraAZrF8JMAM%252CW1T72iDIFCaVYM%252C_%253BmAELRvHLW484AM%252CW1T72iDIFCaVYM%252C_%253BSXHs2vs4ZJo7CM%252CW1T72iDIFCaVYM%252C_%253BOkdvpD5bZs0MdM%252CW1T72iDIFCaVYM%252C_%253B8DGxDo2B7DQhiM%252CW1T72iDIFCaVYM%252C_%253BjrQLEqoJAX4v0M%252CW1T72iDIFCaVYM%252C_&usg=AI4_-kRs7NloUnVz9w452h9mEm3KN-dbsg&sa=X&ved=2ahUKEwjXo4mo_Mv4AhVgD0QIHc0GCkUQjJkEegQIChAC&biw=1920&bih=891
And here is the 2022 Insar (so Far)…
https://www.google.com/search?q=insar+maps+from+Hawaii+younger+than+2022&tbm=isch&ved=2ahUKEwiDiIK0_Mv4AhVkJH0KHRHVDKkQ2-cCegQIABAA&oq=insar+maps+from+Hawaii+younger+than+2022&gs_lcp=CgNpbWcQAzIECCMQJ1DZFlirG2D9HWgAcAB4AIABRogBqwGSAQEzmAEAoAEBqgELZ3dzLXdpei1pbWfAAQE&sclient=img&ei=l8O4YsPgJuTI9AORqrPICg&bih=891&biw=1920&client=firefox-b-1-d
Thanks for the wonderful article, Albert.
A couple of questions:
Is there a thermonuclear reaction generating heat in the inner core?
What is the effect of zero gravity at the center of the core?
There are no thermonuclear reactions (temperatures need to be 10 million K rather than 10 thousand). Zero gravity has no effect because it is an enclosed system. It is like swimming: under water you don’t feel gravity (which is why it is so hard to know which way is up) because you are carried by the water. There is a lot of pressure though
I’ve often wondered if there were fission reactions. Either in the innermost core (fissiles being heavier than iron), or in the lower mantle (fissiles mostly being lithophile rather than siderophile elements, and the oxides maybe light enough to stay up there). The obvious candidates for lower-mantle fission piles would then be the LLSVPs … denser than most mantle rock, also hotter, pretty much as one would expect. But then mantle plumes should barf up some recognizable fission products from time to time, and I suppose we’d have heard something about it by now if that were the case. Instead, mantle plumes seem to like to spout 3He … The inner core would, obviously, contain fission products, and only the heat would escape. And the neutrinos. Are we good enough at detecting those yet to rule out any natural earthbound sources?
We know the nuclear fission rates very well, both on the current and past earth. Going back a few billion years, some reactions were important that no longer are because the elements have decayed. The nuclear decays contributed about ten times more energy for the young earth than it does now. We don’t know where the heat was generated: most models suggest the core is fairly free of radioactives but not all agree. The main decay product is 4He. Whenever you buy a helium balloon, you are buying those decay products. Not 3He: that cannot be formed in decay reactions. Neutrinos from fission cannot currently be detected. But we can detect past radioactive decay from isotopes that they produce.
Another, perhaps even more bizarre, yet not implausible hypothesis for the LLSVPs: what if they are nuclear waste?
There’s no evidence in the geological record for a prior civilization of any kind; and the palaeontological record shows little complex life until a few hundred million years ago. But supposing there had been one. If far enough back, merely refining uranium ore would have yielded reactor-grade material without the need for ultracentrifuges, and in abundance too. There would have been many times as much 235U around as now. It’s not hard to see how this would likely lead to profligate production of nuclear waste culminating in a very messy self-destruction the next time they had a serious war anywhere. The subsequent eons would have eradicated nearly every trace of their works: eroded, dumped out to sea, and subducted. Any remaining seams of artificial stuff would have been transformed beyond recognition: plastics into oil, refined metals into ore veins. The major exception is the longer-lived elements in the nuclear waste. They’d end up in slab graveyards, and being dense, likely sink to the bottom of the mantle, then just sit there, extra-hot and extra-dense, for millions of years.
As for when, exactly, they could have been, it would have to be after metazoans (so max 1GYa) and not so recent as to leave recognizable traces in the fossil record (so probably > 500MYa). An obvious candidate time is therefore “shortly before the Rodinian glaciation”, which would tidily have disposed of the evidence and, combined with their stupid final war, done for much of the complex life and its fossils, including of the intelligent(?) species and its immediate precursors. All that’s left is the seeming “false dawn” of the enigmatic Ediacaran fauna predating the Cambrian explosion, and the LLSVPs …
But most likely the sheer quantity of radioactive waste needed to produce things of the scale of the LLSVPs, and keep them at least somewhat heated for around 750 million years, is far in excess of any plausible amount that such a hypothetical civilization could have produced. The LLSVPs undoubtedly then have a natural explanation, probably related to the core’s patterns of convection in some way. The planet’s rotation would distribute anything heavy at the bottom of the mantle toward the equator, so their positions are partly explained already …
Mount Shasta… looks like a fumarole/steam vent has opened up on the flank of the volcano.
https://youtu.be/mkhtCCqBglI
Its a landslide… rockfalls
Any eruptive activity at Shasta woud be extremely noisy in earthquakes as its a closed conduit system
Not convinced it is just rockfalls, it could be. From the video, there does seem to be ejections of steam. Here is the other video, look from around 3mins into the video. There does seem to be some seismic activity as well.
It is a fire, quite well known it seems just hot easy to see in the day.
If Shasta was waking up it would have been obvious long before. Consider how noisy Fagradalsfjall was, and that was a fluid mafic eruption. Shasta is a silicic volcano, the movement of the magma will cause enormous stress on the surroundings, think of St Helens only that Shasta has been dormant for an order of magnitude longer.
Anyhow, we just have to wait and see…
I agree with the people who are sceptical about claims for an eruption. The rule of thumb is that if in doubt, it isn’t. Too many other things can cause smoke clouds
https://www.alertwildfire.org/region/shastamodoc/?camera=Axis-Weed
Looks like a rockfall, as others have said. St. Helens does the same periodically, sometimes making dust clouds that float over the mountain.
Tallis, any update on Cerro Negro? Still grouchy?
How’s Taal been behaving lately?
Still just steaming and releasing relatively small amounts of SO2 with constant tremor. Nothing special at the moment.
Would love another article from you, time permitting. Even if it’s just to cover recent happenings at some of the more explosive volcanoes lately. Perhaps even touching on those dark horses like Edgecumbe, Davidof, and Cerro Negro. Not that we can expect any action at any of them of course, but kind of just touching on what we know about them, reviewing some of the activity, and implications for the future.
Just a thought. Greatly enjoyed reading some of your past articles.
Thank you! I going to have to wait until we get more information on Chiles-cerro negro.and the current swarm before I make an article.
Has anyone noticed the swarm that occurred around Prestahnukur volcano in Iceland, it started off with a M4.6 earthquake on the 24th June and has so far generated 104+ earthquakes since that event.
It’s way to deep for ice cracking as it was mainly around 5km deep, so it’s either tectonic or possibly volcanic.
It’s a bit weird as I haven’t seen any around there before especially at that magnitude.
That volcano does big lava shields, so for those of us disappointed Fagradalshraun stopped so small, all is not lost 🙂
Prestahnukur, Theistareykir and Langjökull rift Have indeed Perhaps the best capacity of long lived eruptions in Iceland .. they have formed earlier pahoehoe lava shields that Probably lasted 100 s of years in really extreme cases. Basicaly something like an Erta Ale but with more constant shield building that grows up during a humans lifetime.
Luckly there is No big cities near these areras
Would be a lot like Pu’u O’o, only because rifting seems to be minor in the area the conduit isnt compromised allowing the volcano to reach huge proportions. Pu’u O’o was never going to get to 50 km3, even in 2013 magma was starting to accumulate underground elsewhere, and the big quake of 2018 killed any hope of it surviving the eruption downrift.
At Langjokull the shields last for so long they just seem to freeze. Probably a lava geyser that then becomes an episodic overflowing lava lake, abd eventually the lava will just leak out the sides. If the conduit does fail though then we might get a wandering eruption like Lanzarote, the last two eruptions in the Thingvellir graben were of this type. In that case the shield stage is skipped and we just get a years long sequence of cone formation and episodic fast flows, like Pu’u O’o in the 1980s but it keeps going and resets to a new spot. Lanzarote was 3 km3, imagine if it was 10x as large, and 5x as long.
https://m.youtube.com/watch?v=RxrCcmq4kGo
Another Amazing La Palma video showing the deep Basanite that erupted after the staler Tephrite
Defentivly very fluid .. Looking like a river of liquid metal .. but perhaps not more fluid than Halema’uma’u ( But coud be ) its so smooth and runny .. here its competely smooth crust and No Aa signs at all .. coud also be dense and degassed
Sorry, first read for me.
So the Oxygen Fugacity thing pops back up. Yay…. (not).
I ran across the concept when trying to calculate TiO2 production. Ya see, if you work though the equations, You can estimate the sulfur content of a past magma flow from the TiO2 percentages. A big source for error is Oxygen Fugacity. It turns out that Oxygen can interfere with TiO2 production and skew the estimate. The activity of Oxygen is quite variable depending on the environment. It is dependent on prevailing temperature and pressures.
It kicked my arse for a bit then I gave up on using that technique because of the chance of having to defend crap data.
(If your data is shit, so are your results.)
https://www.ina.fr/ina-eclaire-actu/video/i07281217/l-aventure-du-nyiragongo
Nice video of Nyiragongos lava lake .. showing how low the viscosity is of a Nephelinitic melt .. But its probaly the same as hot Thoelitic basalt in Hawaii and Iceland, Despite Nyiragongos extremely low Sio2 content, because temperatures impacts viscosity almost as much as Sio2 .. in a hot normal basalt the Sio2 polymerisation is broken down
But Nyiragongo is extremely sillica undersaturated and retains lower polymerisation at any given temperatures compared to a normal basalt
I think that a truly primitive nephelinite is probably more fluid than a tholeiitic basalt. Nyiragongo’s nephelinite has evolved a little from fractional crystallization, I remember from when I plotted them. The Toro Ankole volcanic fields north of Lake Edward erupt primitive nephelinites together, maybe the difference with Nyiragongo is not too great, but I expect those magmas are probably more fluid. This is due to less polymerization, they are undersaturated in silica and aluminum, while richer in practically all other elements compared to other basaltic varieties. And I’m not sure they are any colder than tholeiites. The La Palma basanites were reported to have temperatures of 1150-1200ºC which is in the same range as Kilauea tholeiites. So more alkaline doesn’t necessarily mean colder as far as we know, but it does mean less silica and less aluminum, which is less polymerization.
You find nephelinitic volcanic fields were you least expect. For example in Oahu, or some old not sure if active volcanic fields in Honshu and Iran, and there are also the Urach and Hegau volcanic fields in Germany. Nephelinite eruptions are very rare though. Apart from Nyiragongo and Visoke I’m not sure if there has been any historical nephelinite eruption. And only Visoke was truly a primitive nephelinite (I think, don’t remember very well from when I plotted it). The Mugogo eruption of Visoke in 1957 was minuscule, lasted only 42 hours and made a 1 km long flow. Very rare magmas.
The first phase of the eruptions on Lanzarote was apparebtly nephelinite, little remains on the surface. These flows were erupted at a high rate much faster than most of the rest of the eruption, flooding a huge area of the island very fast. Is also unusual in that the eruption of this lava was from only one vent to begin with, not a curtain of fire.
The eruption though seems to have induced massive decompression in the mantle because the magmas rapidly became alkaline basalts and then even tholeiite basalts, the only such eruption in the Canaries historically to erupt such lavas.
Yes, it almost reaches the nephelinite field. Here is the TAS diagram for Lanzarote taken from EarthChem:
There is a magma series extending from basanite to tholeiite basalts, that is the seies that the Timanfaya eruption followed, starting as a basanite and ending as a tholeiite. Oahu is probably similar it also spans a large range from some of the most silica-undersaturated nephelinites in the planet to alkali basalts. The eruptions probably start as nephelinites and as they go on they become gradually more tholeiitic, if you kept extending the series you would reach to the left end (the primitive end) of Koolau shield volcano fractional differentiation series.
TAS diagram for Lanzarote (EarthChem):
I don’t think there exists any nephelinite caldera either. There is Colli Albani, but it seems the parental magma of Colli Albani is a tephrite or phonotephrite, not nephelinite, it gets into the foidite-nephelinite field through fractional crystallization because of how the TAS diagram is drawn where the foidite field reaches up to higher silica contents when the total alkali content is higher than 10%. So I don’t think that one counts.
Colli Albani magmas range from 44 to 50% SiO2 as they evolve from tephrites to foidites due to fractional crystallization, which is very low, maybe the most silica undersaturated caldera in the planet (that can be considered active). However the Oahu volcanoes have erupted as low as 35-40 % SiO2 sometimes, although it has a very wide range. Katunga in Toro Ankole erupts with 35-38 % SiO2. Urach in Germany erupts 34-38 % SiO2.
Mugogo erupted about 39 % SiO2, and Nyiragongo which probably has a parental magma similar to Mugogo evolved through fractional crystallization to 37-40 % SiO2 or so. So even Mugogo and Nyiragongo do not really belong to the most ultrapotassic, silica undersaturated series of magmas in the planet, which Urach, Katunga, or Oahu represent.
Here is Urach in a TAS diagram, actually many of the samples fall outside the diagram, to the left, because of being too silica undersaturated:
The TAS plot of Urach:
Also, as far as I know those silica undersaturated magmas are extremely explosive, always making maar-diatremes. Or at least Toro Ankole and Urach are seemingly made up of maar-diatreme volcanoes only.
I guess eruptions like those of Urach or Katunga are extremely rare, short-lived, small-sized, and heavily explosive. Although this is a guess of mine on limited information. But nonetheless it is an interesting issue. These would be some of the rarest eruptions in the planet, at least from a chemistry point of view.
Tholeiite basalts like those of Hawaii or Mid-Ocean Ridges are low-gas and thus little explosive. Fractional crystallization turns the magmas more explosive by increasing the gas and viscosity, needing much bigger conduits to carry the sluggish melt. But it also works the other way around, less silica (more alkaline, in reality more every element that is not silicon or aluminum) means more explosive due to increasing gas content.
I do remember seeing a close up of a repeating breakout at La Palma, it might actually be the same one as reported in the paper linked last article, dated to November 25. Unfortunately there is nothing saved showing that flow in daylight, but the speed at which the lava moved was incredible, much faster than anything from Fagradalsfjall. It looked just like the old videos of carbonatite lava at Ol Doinyo Lengai, except this was in ‘real’ lava and of huge scale. Was incredible to watch.
There is also a video of a cone collapse about 20 dats into the eruption. The collapse starts as a pyroclastic flow complete with a phoenix cloud and everything. Immediately afterwards the ponded lava in the crater floods out and it forms a secondary fountain over an obstruction in the flow. The video is very overexposed but probably shows the fastest flowing lava ever caught on camera, it moves probably at least 100 meters in a few seconds.
The fluid lava also showed up immediately unlike what was thought at the time, the viscous flows seen earlier in the eruption was an illusion because many of those flows came from fountain fallout or were the cone itself basically flowing downslope. The first days also were more explosive, effusion became more important about 2 weeks in. Once efficient degassing was able to happen from the upper vent the liquid lava seemed to flood out of every crack it could.
The conversation got me watching old La Palma videos, IGME has a lot of great videos with incredible quality. The behaviour of the lava is mesmerizing. Certainly very fluid. This was basanite, between the fluidity of basalts and nepelinites (presumably).
Bermuda also produced insanely sillica undersaturated in its postshield dying phase.. Bermuda Nephelinite cap range as low as 35% Sio2 they are also known as ”Bermudites”
Interesting. These magmas occur in many places really, but usually in very little amounts forming low-volume volcanic fields.
Exactly because their origin of very small ammounts of partial melting so only very little Nephelinite is produced
Nyiragongo is by far the most massive Nephelinite volcano on the planet .. and is quite unique with that … with the other Nephelinites being mostly monogentic cinder cones and maars
Special conditions must have allowed the formation of extra ammounts of Nephelinite under Nyiragongo to allow it to become a highly active central volcano
There is one other oddball it seems, Ol Doinyo Lengai. Ol Doinyo Lengai has some nephelinites that are very similar in composition to Nyiragongo’s in fact possibly more silica under-saturated. They both have a concentration of samples in the 35-40 % silica, 10-12 % total alkali range, which is the current composition being erupted by Nyiragongo. So Ol Doinyo Lengai and Nyiragongo seem to have erupted similar evolved nephelinites. I’m not sure if Nyiragongo is into that anymore though.
Here is Ol Doinyo Lengai:
Here is Nyiragongo:
Interesting that there are rhyolite samples from Nyiragongo. Maybe granite xenoliths that were partly remelted.
Also I remember reading that the 2007 explosive eruptions from Lengai were nephelinite. There might have even been a small lava flow.
Yes, those are probably samples of granite xenoliths, I did not filter out other rocks types or xenoliths in my search. Any sample that falls in the general Nyiragongo area will have made it to the TAS plot.
Remember reading somewhere that many flood basalts are preceded by alkaline volcanics, comparitively of small scale but in reality that would mean something quite big in absolute terms. Just one of many reasons to think the area is going to eventually become the next LIP.
Technically speaking, that would mean there is an active LIP today, because a far future observer would take the early volcanics as stage one before the main phase. Nyamuragira might be the actual volcano itself already, would make sense to use an existing outlet over creating a new one. Nyiragongo is an open vent and very strong degassing and heat source but doesnt seem to actually erupt a lot of lava, Nyamuragira has more frequent and usually much bigger eruptions just isnt quite so open at its summit, well it wasnt before 2014.
You are going a bit over the top here.. Nyiragongo is active but not major, it is not at either of the ‘bulges’ in Africa, and for a LIP you do not want a single volcano: those are rifts hundreds of kilometers long. Eventually Earth will have another one, in 10 or 20 million years when any current volcano is long gone.
Not sure every LIP is the same, some are on rifts, but some seem to be centralized. One can look at all of the plume volcanoes today, Hawaii, Galapagos, Iceland, they all erupt lava that is basically identical (and the same as most LIP basalt) but morphologically they are very different.
Virunga also is actually quite elevated, by more than 1 km above the expected altitude. Alkaline volcanism is also not expected to be so productive or so hot, and yet Nyamuragira might be the second most productive of all volcanoes behind only Kilauea long term, and at multiple points since 2000 has been the most powerful heat source of any volcano. Nyiragongo doesnt erupt a lot of lava but it also comes close to Kilauea in thermal output as well as in SO2, there is a lot of heat down there but the mantle has not yet began to decompress and melt at a shallow depth. Given that the Albertine rift is otherwise basically non-volcanic it seems something is definitely going on there.
On another note, there is a volcano called Chyulu Hills that is located on the Kenya bulge, and is a mafic rifting fissure. Its lavas have also become less alkaline with time, suggesting greater degrees of melting. Ethiopia saw a LIP when it opened, along with the Red Sea, the rest of the rift valley has lagged behind but seems soon to follow suit.
Iceland and Hawai’i are not LIPs. They have formed impressive mounts, but have taken far too long for it to be called a LIP. LIPs seem to form within a few million years and probably much faster than that.
Ethiopia rifted 30 million years ago. The other two arms of the triple junction progressed, but the Ethiopia rift did very little. I would not call 30 million years delay ‘soon’. (Mind you, our railway company would happily do so.) Africa is full of extinct or mostly extinct rifts. Why is Africa doing so poorly (or keeping together so well), ever since Madagascar and India left? The reason must be the lack of subduction pull. There was a pull to the north, from Asia, which helped the red sea develop. But there is very little pull east- west. So the east-west spreading rates are low and inconsistent. There was a mantle plume under Africa and Arabia 30 million years ago and we may still have lingering heat from that, but there is no convincing evidence for other plumes, just shallow heat. Or this area may benefit from a bit of rotation (differential spreading of the east and west african rifts) which provides a bit more pull here. The dead sea is like that: it developed because of rotation of Arabia, but did not grow further for lack of pull. To really get going in Africa, you will need a subduction zone in either the Indian or Atlantic oceans (preferably both) which pull Africa in the east-west direction. Perhaps in 50 million years. At the moment, rotation is the main driver of rifting in Africa but rotation is much easier on the edge of a continent than in the middle.
Virunga has very dangerous volcanoes and you are right to call attention to that. Speculation on where a future LIP might happen is also fine for VC. Going for imminent world doom and assigning a particular volcano to it requires much stronger evidence than you present. Why not Bagana, growing up in 300 years, Erebus with a perpetual lava lake, Etna, or New Zealand with its regular big booms? Or if you want a flood basalt in Africa, why not go back to Afar? The Stratoid formation there is perhaps only 2 million years old and it comes close to being a flood basalt. It was triggered by rotation of Ethiopia
As far as I am considered the TVZ is an active silicic LIP. It is not exactly on the huge side but is very active for the area that is covered. Yellowstone I also consider to be an active LIP, the same one as once spawned the Columbia River basalts and still going today. Trying to make a post about it so that is all I will say on that subject 🙂
I also think there does need to be a distinction between a flood basalt (basically Olympus Mons on Earth) and LIP. Hawaii is an igneous province that is indeed ‘large’, and Hawaii has also formed in a relatively short time, the Big Island began forming less than 1.5 million years ago and is over 200,000 km3. The Columbia River basalts (which are always considered a LIP) was probably less voluminous than this, and even the most intense phase (Grande Ronde basalt) was still over a longer time period than Hawaii has formed in. Keep in mind that is only for the Big Island, the number at least doubles if you add Maui Nui which began forming less than 4 million years ago. So half a million km3 in 4 million years. That is not as fast as the Deccan Traps but very respectable. Adding Oahu and Kauai might even bring it up to over 1 million m3, in 7 million years. That is not really all that far off of the Deccan traps, within a factor of 3. Hawaii though doesnt really erupt on a scale too different from volcanism elsewhere, it is a gigantic ‘normal’ volcano.
What I was also hinting at is that we tend to imagine flood basalts as being like Iceland because that is where Laki happened and probably until 2018 that was the best concept most people had. But in reality there could be flood basalts that behave more like Hawaii or Galapagos instead, with only eroded remnants it is not exaclty easy to tell, and 2018 showed the world that Hawaii can also do eruptions of high intensity and large volume. Given that most cases of plumes surfacing would be statistically unlikely to do so under an existing divergent boundary, it migth even be more likely the LIP resembles one of the other two locations, or even that a combination of features exists.
I think the eruption style should be considered. The Columbia flood basalts did individual lava flows as large as 4000 km3. Bushveld had a 1,000,000 km3 magma chamber. Afar probably sent giant dikes all the way to the Mediterranean Sea, as attested by radiating graben systems that reach the Mediterranean Coast:
https://cdnsciencepub.com/cms/10.1139/cjes-2016-0019/asset/images/large/cjes-2016-0019f9.jpeg
As I’ve mentioned before, Martian volcanoes, which are like LIPs, usually have dikes that reach 2000-3000 km long, and tend to produce lava flows which are a few hundred kilometres to a few thousand kilometres long. Not to mention that intense fissure eruptions in Mars, Venus, and the Moon excavate massive canyons into the ground through thermal erosion.
That is why no present volcanic activity on Earth should be compared to LIPs, I think, the eruption style is too different. Kilauea, Nyamuragira, or the Snake River Plain do not produce any eruptions that are beyond the scale of those in every other volcano in the planet.
The size of individual structures it’s what telling about a LIP I think. How long a dike is, or how long a lava tube (Alba Mons has lava tubes 900 km long, as do other Martian volcanoes), how voluminous a flow or magma chamber, or the size of a lava channel. In those things LIPs, and extraterrestial volcanoes for that matter (Io, Mars, the Moon, Venus and Mercury) stand far above typical Earth basaltic eruptions.
That is what I mean though. Hawaii and Iceland are Large Igneous Provinces, they are not flood basalts, but the name LIP doesnt exactly distinguish. I think any large mass of igneous rock that is not average cratonic granite or average seafloor basalt should be considered a LIP. So Hawaii and Iceland are not flood basalts but are LIPs.
I also think a place capable of producing repeated VEI 8 from a single center needs to be considered, there are several such locations active today, and the Yellowstone track definitely need mention here, going on probably 30 eruptions of high VEI 7 to 8 (‘supervolcanoes’). Different mechanism again, so perhaps 3 ways. Maybe even many more as you could get hybrids of the above.
Quote: “These Large Igneous Provinces (LIPs) are best preserved in the Mesozoic and Cenozoic where they occur as continental flood basalts, volcanic rifted margins, oceanic plateaus, and ocean basin flood basalts.” (http://www.largeigneousprovinces.org). LIPs are indeed meant to be the equivalent of flood basalts. They are classified to area (not volume which would be very hard to measure for an ancient and eroded LIP). There isn’t a strict limit, I believe, but they are typically 0.5 million square km or more, at least originally. Say 500 by 1000 km minimum. Virunga does not get near. You’d need to cover half of Tanzania to qualify.
‘Volcanic rifted margins’ is a bit ambiguous here. The Red Sea and Afar regions would fit that and are still active. The east coast of Australia in a much older example, yet also a still active one. Seems a very different thing than a flood basalt as Hector describes. Also seems a bit strange to include that but not Iceland.
You are missing the point. The Afar flood basalt is the Red Sea rifted margin. As Hector pointed out, the outpourings extend almost to the Mediterranean. Rift flood eruptions are not at the center but on the edges of the rift. Iceland does not have such a rift and probably never had: it is too small. You could have pointed out that Africa does have such a rift and Virunga is located along it. Virunga itself is far too small to count, but if the rift for any reason develops into a real one, then a flood basalt in central Africa is possible. It is conceivable that the Hawai’i plume started with an oceanic flood basalt. I think it started on a rift in the central Pacific. But that basalt would now have been subducted. For a flood basalt you are looking at a fast event. A million year is the typical quoted number but the actual eruption spikes seem to be much shorter, one or two hunderd thousand years. That is seen in the Deccan and Siberia traps.
Actually I am not missing the point, there isbt abything like a flood basalt on Earth today. But there are places active today that fit under the term of ‘volcanic rifted margin’. The Red Sea was not there when Afar was created, the plate boundary seems to have been created by the event but not what started it. The volcanism on the Arabian coast of the Red Sea has been continuous sibce then, of low intensity but it is not the same as mid ocean ridge volcanism. It is the sa e thing where Greenland was erupting for a long time after the opening of the North Atlantic, which began with flood lavas too. Iceland is sort of a continuous transition from that just without the intensity of a flood basalt.
I did also notice, the actual map on the LIP Comission website, it does include Hawaii as a LIP, along with all the other volcanism in the Pacific and Indian oceans that was not erupted from the divergent boundary. So they dont actually specify that LIP = flood basalt, just that most LIPs in the older record would have been flood basalts as they last longer in time as a structure that is recognizable.
How is that for confusion. Their table of LIPs has Columbia as the youngest event and does not list Hawai’i. The map they show is much older than the book it advertises and does not agree with their own map of ‘recent’ (less than 500 million year) events which comes from that book (below) – and which does not list Hawai’i. Wikipedia also does not list Hawai’i as a LIP but does have Iceland – but as a remnant of the flood basalt at the splitting of the Atlantic which in fact long predates Iceland. You may have noticed that the LIP commission lists Brisbane as a possible flood basalt of uncertain age, with as caveat that the detection of dikes remains uncertain.
Probably to avoid the confusion, and not give a specific example, flood basalt events should be called Traps. Many already are, and it is a distinguished term from ‘flood lava’ whicb can correctly be referred to some modern eruptiobs that were not trap formations or even necessarily large.
What I have been proposing is that not all LIPs are trap formations, some formed over longer time periods. Iceland really has a good case to be called a LIP even if it lacks the scale of volcanism associated with a trap formation. I do wonder if there is some subconscious but significant perception that events of this magnitude are a thing of the past, like how dinosaurs are always imagined as indestructible monsters that attacked everything that moved rather than the living animals they were. The whole ‘things used to be bigger’ idea.
Batholiths also need mentioning. Active batholiths like the Andes have huge magma chambers that feed whole clusters of volcanoes, only difference is these are rhyolitic not basaltic. Even if one distinguishes a VEI 8 caldera as not being a LIP scale event, there are magma chambers of an order of magnitude larger than that in the Andes, right now.
Problem is that LIP, trap, and flood basalt, are generally terms that have been used with the same meaning. I do understand what you are saying in that Iceland, Hawaii, Galapagos too, have massive volumes of basalt, although erupted over a longer period of time than LIPs. Of course they are a thing of their own, calling them LIPs would be confusing. Maybe there is the problem that the acronym stands for Large Igneous Province. If you take the meaning of those words literally any large area of volcanism could qualify. Why not the Central Asia region of volcanic fields that extends from Baikal to Japan, or the East Australia Volcanic Province, or any large volcanic arc for that matter. Problem is, that is not what the term LIP was meant for. I do agree that volcanology is need for a couple of new terms, but the term LIP is taken I’m afraid.
The criteria they give in http://www.largeigneousprovinces.org/ for a LIP is the following:
1 = mafic magmatism > 100 000 sq. km. (or >100 000 cu. km.) and short duration (<10 m.y.). The key types of magmatic units used for this estimate are coded as follows: v= volcanic rocks (mainly flood basalt sequence or its erosional or deformational remnant), s= mafic sill province, i = layered mafic-ultramafic intrusion province, and d = mafic dike swarm.
2 = linkage to present-day hotspot.
3 = giant radiating dike swarm.
I do agree that finding giant dikes is a key requirement. Giant dikes reflect the potential of volume of eruptions as well, given that dikes and eruptions are both forms of allocating magma from the asthenosphere or a magma storage to somewhere else, and their size will be controlled by the storage volume or the supply from the asthenosphere of a given volcanic area. The size of dikes and eruptions possibly measure the rate at which magma is being released from the asthenosphere, although this last thing is an speculation of mine. In fact I suspect some of the giant dikes I've seen in Mars may have formed over a very long period of time growing with the supply from depth, the reason is that these giant dikes often have long-lived shields as vents.
The size of very old lava flows is difficult to know, dikes are a bit easier, it is easy to establish the length and width of a dike at least.
You might expect that dikes on Mars will be about 3 times longer than equivalent dikes on Earth. That is because the level of neutral buoyancy is three times deeper on Mars, so under the same angle it takes that much longer to reach the surface. (This is of course a very rough argument as many other effects come into play). A dike from a single volcano may reach ~100 km on Earth, and a LIP dike may reach ~1000 km. Multiply by 3 for Mars.
The main reason I think Iceland should at least qualify regardless is because it actually has a qualified direct counterpart, Kergualen plateau. Back during the Cretaceous it was basically the same as Iceland today, perhaps even more active, and it formed in a similar manner from a hotspot under a ridge. It also is always considered a LIP despite lacking a giant dike swarm, it is even a poster child of the term in many cases. One section does seem to have been a proper trap formation, but the other parts were constructed slowly, in light of this it seems a bit pedantic to exclude Iceland from the LIP list.
Kergualen also is still active, actually Heard Island is nearly constantly erupting. So technically speaking, there is a currently erupting LIP 😉
Nyiramuragira is growing into a behemoth .. and gone shield building after 2016 almost.. with pahoehoe fillings in its summit .. Both volcanoes may gets some lava stolen ny passive rifting as well .. Althrough rifting is much slower than in Iceland and allows more lava to reach the surface
No Erta Ales, Nyiragongos or Nyiramuragiras in Iceland probaly rifting is too fast I guess
Really superfast oceanic ridges dont form central volcanoes.. or more like long Fissure ridges feed by a melt lens that spanns 100 s of km
Nyiragongo seems to get its magma stollen by rifting, like in 2021. Nyamuragira doesnt seem to rift at all though its eruptions dont align with the rift valley. Perhaps that is why Nyiragongo isnt a shield volcano, only the most gas rich magma can erupt so has made an oversized pyroclastic cone that leaks during rifting events.
https://www.volcanodiscovery.com/nyiragongo/news.html
Nyiragongo is reforming its summit lava lake
Nyiragongo and Nyiramuragira is the only place in the Albertine Rift that are that hyperactive and the only place outside Erta Ale thats hyperactive in Africa. Virunga is quite productive and the high production started relativly recently. Albert I think we have an emerging mantle plume there.. thats Chads point .. the intensity of the activity
Hennes a nice video about the initial investigations of the canaries eruption by my favourite Uppsala professor.
https://youtu.be/-Fsae0_PAzM
Anything interesting going on at La Palma?
Source: https://www.ign.es/web/ign/portal/vlc-area-volcanologia
Chiles-cerro negro will probably have 50,000 earthquakes this month, and the quakes are seeming to get more concentrated near the Cerro negro peak. Uplift has started to accelerate as well.
It would be a most unusual situation to have a volcano that has been dormant for so long erupt. I’m curious as to what would happen in that case.
If this volcano does erupt.I think it’s going to be a big one. Not only has this large volcano has been long dormant, a couple of other volcanoes evidently are part of the same system and We’ve had very deep LP and VLP quakes down to 35 km below the surface.
None of the past swarms have started with this level of intensity, and I am very interested in what’s going on and I will write an article after I get more information.
Wont know until it happens, but this could be a great opportunity to test the theory on ignimbrite formation. Hunga Tonga suggests these may form very fast, but then there is also the water at play. If Cerro Negro does a blast of comparable intensity it suggests such eruptions dont need water in abundance to happen.
That being said even if an eruption happens it might not immediately go boom. Tambora was erupting for several years before 1815, and it seems the geological record shows Cerro Negro to be generally effusive, so will probably not exactly go nuclear out of the blue.
The swarm at Bardarbunga is continuing with a M3.5 at 2km depth this morning
Since the elevation of Bardarbunga is about 2km, I presume the quake was 4km below the surface. Big difference if my presumption is correct, because if not, if fits Carl’s 2km/2M really-worth-watching-rule.
Nice videos of Fagradalshraun…Thoelitic action at 1240 C same temperatures as Kilaueas shallow magma stoorage. Fagradalshraun 2021 Hows How high temperatures affects a normal sillica saturated Thoelitic basalt, temperatures lower polymerisation alot for soure .. probaly as fluid as Nyiragongo I guess
https://m.youtube.com/watch?v=kejICJptSA8
https://m.youtube.com/watch?v=3eV0qQ0Cm_A
https://m.youtube.com/watch?v=eedpfTFjWac
https://m.youtube.com/watch?v=ZmBsWFA86gs
https://m.youtube.com/watch?v=WpxsBFgxpgE
https://m.youtube.com/watch?v=9l2hPu_6aVU
Comments with series of links will always be intercepted by the spam deamon. Best to limit yourself to no more than two links – admin
Very low viscosity for Fagradals with fast flowing narrow rivers and standing waves and moving waves and yellow white even in daylight for hottest exposed surfaces. Many Fagradals flows also appeared To be flowing turbulently in the channels and vent lake. I guess similarly low viscosity to La Palmas deep Basanite
Temperatures are very good at removing the sillicate chain polymerisation
What was the viscosity of Fagradalshraun in PA.s ? Hawaii for example can go well below 10 in rare cases .. Fagradals also seems to be a highly fluid ”lava foam”
For Hector.
Not sure if you have a better map, but this is a quick one I made that shows the two grabens up on the Libyan Plateau that you said were probably radial dikes from the Afar LIP. While not completely implausible, the distance does seem, well, very extreme… Afar was not a really extreme LIP, it seems the modern extent of the basalts is not too much less than what it was originally, and the province seems to have not abruptly stopped but gradually slowed down to the modern configuration with little change of activity except for absolute scale.
Africa also has some really thick crust, enough that the rift has still not completely broken it in 30 million years. I doubt dikes would be able to travel that far through a craton. Even if that is an incorrect assumption it seems likely there would be much more visible effect given the event was geologically recent and Africa has not really changed much overall in that time. I expect the Nile would have followed for example given it starts in Ethiopia.
It is not widely known but Afar had two flood basalt episodes. The first was mainly extrusive maybe a shield building event like Martian or Venusian shields, which deposited large amounts of basalts across Ethiopia and Yemen about 30 million years ago. No giant dikes are known from the first episode. Following this event there were one or more pulses, particularly around 26-23 million years ago of mainly intrusive activity which consisted on the formation of a series of giant dikes that propagated towards the Gulf of Aden, making the Ogaden dike swarm, and also towards Kenya, but mainly along the Red Sea towards the Mediterranean. There were minor amounts of lava erupted, however some of the dikes did erupt in El Cairo area:
https://www.researchgate.net/publication/277027020_Integrated_outcrop_3D_seismic_and_geochronologic_interpretation_of_Red_Sea_dike-related_deformation_in_the_Western_Desert_Egypt_-_The_role_of_the_23Ma_Cairo_mini-plume
https://www.researchgate.net/publication/283642026_A_major_dyke_swarm_in_the_Ogaden_region_south_of_Afar_and_the_early_evolution_of_the_Afar_triple_junction
https://cdnsciencepub.com/doi/10.1139/cjes-2016-0019
“From ∼26.5 to 25 Ma a new phase of volcanism began with the intrusion of a dike field reaching southeast of Afar into the Ogaden. At 24–23 Ma dikes were emplaced nearly simultaneously north of Afar and reached over 2000 km into northern Egypt.”
Some of the grabens made by the dikes are still intact preserved by the desert climate of that area. There are the 2 you have mapped but also some of them can be seen along the Nile, near Asyut:
There are many visible along the Arabian side of the Red Sea, near Harrat Uwayrid. They are best seen in topography:
https://maps-for-free.com/
In Mars it is sometimes seen for volcanoes to have separate shield building and diking events. For example Alba Mons did its shield building sometime 3 billion years ago, during this time it didn’t produce any flank vent. About 100-200 million years ago or so Alba reactivated, roughly during the same interval Olympus Mons was constructed and the Tharsis Montes reactivated, during this time Alba produced a series of circumferential dikes (cone sheets) around the caldera, and radial dikes extending ~2000 km north and south, probably hundreds of them if I had to guess. These dikes cut many large craters that had impacted on Alba shield building lavas during the long dormancy. Interestingly very few of these dikes erupted, and it was usually small patches of lava into low areas, like craters, or catenae. Olympica Fossae was one of the few significant eruptions of this episode. Venusian giant radiating dike swarms are often non-eruptive too. The CAMP too was like this, it did dikes as long as 3000 km, one of them cuts across the Iberian Peninsula, and some giant sills are known too, but there is comparatively very little effusion. So it is something that does happens, and there is somewhat of a duality in LIPs, some more effusive, other more intrusive, or usually it may come in phases.
Regarding the dikes being non-eruptive, it is probably a matter of how tall the volcano is above the surroundings. dikes loose pressure along their length, so they can only erupt at elevations lower than the summit of the volcano, the greater distance from the summit the lower they will be able to erupt. There is the example of Taal that has sent three huge dikes outside the caldera in historical times, none of which could erupt. Taal is depressed below the surrounding area so dikes never have the pressure to erupt. Hawaiian volcanoes have a relatively constant slope along their rifts because of this, because magmatic pressure is lost over distance. So the rift axis has a slope that matches with the rate at which pressure decreases.
Similarly some tall Martian volcanoes like Elysium Mons or the Tharsis Montes were very proficient in erupting from their flanks or far away, and the elevation at which the dikes erupt decreases with distance. Volcanoes with relatively little relief like Alba Mons or Syria Planum did mostly non-eruptive dikes. Some sort of elevated central edifice is require to push lava to the surface at large distances from the summit.
This might be a bit unrelated to this discussion but when I heard the word “Martian volcano “, it just clicked. This might be supposed to be in the VC bar but this would be an exception.
Paint this picture: a moderate flood basalt 35 million years ago by hotspot underlying a craton that hasn’t experienced any geological activity in billions of years in a continent moving about 1.6 cm/yr in Earth-like conditions. 5 million years after that, a mega-volcano forms as the continent begins to slow to 0.6 cm/yr and overtime forms a massive ice cap covered volcano that stands about 9 kilometers in height and about roughly 600 km in width. The area around it sinks with it as it grows. Is this sort of thing possible?
Volcanoes like those do exist on Venus and Mars. As for Earth I’m not sure it has ever had LIPs that were so tall. It is hard to know but Afar originally would have probably looked as a cluster of shield volcanoes and calderas ~4 km tall and spanning an area ~1000 km across.
I kinda know that the Afar isn’t really suitable for a mega volcano like that. The area at the time would’ve been too unstable to support the weight of this. What I was thinking is a megavolcano say forming on a very stable setting (I.e. a really thick craton, like the North American craton), but this is more like a what-if rather than something of reality. I asked those questions because (as a worldbuilder) I was thinking about making a massive mega-volcano on what is pretty much a supercontinent on 30 degrees latitude. I am just sort of validating the realism of that.
I mean if Hawaii was where Yellowstone is now that might exist, Hawaii is 9 km tall. It would probably be more like 7 km if not in water but still most definitely the tallest freestanding mountain. It is kind of mind boggling that basically every other mountain on the planet would not even reach the sea surface at that location, and then not only does Hawaii do that, but does so over an area of about 4500 km2 and then has two mountains that go to 4+ km on top.
I think though, 10 km could be a hard limit on Terran mountains, and maybe even 9 is pushing it. Everest is probably not far off the true limit. Venus has mountains up to 11 km, and gravity slightly weaker than 1G. I did hypothesize in a far future event where Pangea 2 is constructed, it could involve collisions of two mountain ranges. Specifically, in the area between the Americas, a subduction zone will probably form on the west Atlantic forming a new mountain range, and then the Americas will also move together, turning Mexico into a double collision from both axis. I imagined an unstable cobfiguration where the peaks were going into the stratosphere.
https://www.deviantart.com/el-d0rito/art/Atlantis-200-million-years-in-the-future-596352470
Yes this is actually my old account.
Well, it is indeed a freestanding volcano and, other than possibly big lakes and rivers around the depression, it has no water around it. The eruptions from this volcano would go on many volumes, from small spatters all the way to larger-than Laki eruptions that pour lots of lava over tens of kilometers, creating the volcano over a span of around 30-35 million years (assuming the hotspot is just as powerful as the Hawaiian hotspot), but, it also needs support.
That support comes in the form of a very ancient craton, with the lithosphere it standing on around 300 kilometers thick (I think, or more), but the volcano would have a slope gradient less than Olympus Mons. There lies a bit of an issue. Would the hotspot create such a volcano, or would it create a rift and make many smaller ones… or will the hotspot, widespreadly over a large area, thins the lithosphere so much the volcano may sink further, making less tall. It is quite a curiosity of mine since I have read the infamous “what if Olympus Mons was on Earth” and I was quite speculating on that.
https://imgur.com/1xTGTnK
map of the volcano I’ve created. The lines are pretty much the dikes and the faint blue-ish white is the glaciers themselves. Scale is a bit harder to see.
Seems really cool to me. I would keep that volcano even if the physical viability turned out to be somewhat questionable. It is a imagined planet after all. Imagination can try to adequate to reality, but there can’t be perfection.
That’s the thing. I always question my own creations. Something like a shield volcano upon a hotspot and creating my own I will be confident of because there are a lot of papers that I could read off of to put a sort of “ruleset” to it, but a mega-volcano like this? In Earth-like conditions?
Not many papers pondering about the idea of a near-Olympus Mons copy forming on Earth other than speculation from the smaller volcanoes. I need to see what is wrong with this volcano and any papers so that I could get a idea of what I may have to do. I need constructive criticism.
To be fair regarding my original hypothesis in the other comment, the first stage of Afar seems not too far beyond what I think Virunga could become. I imagine the second stage was after the onset of spreading, allowing for dikes to move along the rift that would become the Red Sea, the earlier stage probably preceded any movement and maybe is what finally broke the plate.
I did some research on the subject, the Kenya rift is a separate rift that only recently (less than 2 MYA) connected to the Ethiopia rift. Kenya never had a tholeiitic flood lava event like Ethiopia, it was not a proper LIP in the sense of being like a mega-central volcano. But it did have a large flare up of alkaline volcanism of almost flood basalt scale, the Samburu volcanics, and that is what I think could well happen within the next million years at Virunga and the rest of the Albertine rift which seems to have been completely inactive until recently, not exactly a place to expect a volcano like Nyamuragira. Whether that becomes a full blown traps event maybe is a different matter but seems a reasonable possibility, at least it is a 50/50 going from the other parts of the EARV.
Yes I guess it is not too simple, the EARS has seen multiple events that could qualify as LIPs. The Amaro-Gamo tholeiitic basalts had been erupting since 45 Ma, in Ethiopia, thus preceding the climactic phase. Sometimes the Amaro-Gamo basalts are also referred as flood basalts, although I’m not sure what is the justification for this. Then there was the main traps phase where eruption rates peaked at about 30 million years ago, large shields like the Simian shield volcano grew, together with a bunch of calderas that did VEI 8+ eruptions. But then it wasn’t until 25-23 million years ago that the main phase of giant dikes took place and produced 2000 km long intrusions along the Red Sea. And there there still was the Samburu/Kenya flood phonolites, that erupted 50,000 km3 of phonolite lava in the short period of 14-11 million years before present, making two large shields 3-4 km tall. So you may be right in that the boundary of what is a LIP or not may seen somewhat unclear at times.
That is why I proposed that the name traps be used for the extreme stuff. LIP can include traps, but probably in most LIPs the volcanism can be more moderate too. The Amaro-Gamo volcanics was probably not too different from Iceland. The traps phase then took place, and then the shields formed overtop of that, or forming calderas instead over in Yemen. This stage would not have been any more intense than Hawaii or Iceland, massive scale stuff still but not extreme. But this is still a LIP.
I know LIP was made to talk about the same thing as a trap formation, but it is much better and makes more sense as a blanket term. And if Iceland isnt a LIP I actually dont know what it is, certainly it is not just a standard piece of ocean crust, and all the old submerged oceanic plateaux that are what Iceland will become one day, are unanimously referred to as LIPs…
One of those things it seems where the word was defined on a thing that was not well understood or well defined either. And, as I think, it it a case that we cant have a modern example for some reason.
“And all the old submerged oceanic plateaux that are what Iceland will become one day, are unanimously referred to as LIPs…”
That is a fair argument. If everyone is calling Kerguelen, or the Mid Pacific Mountains as LIPs then why not Iceland that is basically the same, morphologically at least.
Kerguelen is a funny one. It is no longer thought to be associated with a flood basalt, but with a very long lasting eruptive phase. ‘Long’ here means 30 million years. The recent volcanics (Heard Island) seems unrelated to the Kerguelen volcanics. A recent paper called it ‘unique’. Other oceanic LIPs did not form in this way. Quite a lot of speculation on what is special about Kerguelen. One of these invokes a very slowly northward drifting spreading centre. There are some similarities with Iceland, but Iceland is much smaller than Kerguelen. (If you take the old definition of a LIP needing 1 million cubic kilometers, then Iceland is still a factor of 2 too small to be one.)
Are you sure Iceland is too small? Just a quick calculation even the height above the seafloor is about 1 million km3, and the evidence suggests the crust is a lot thicker than typical ocean crust so this is a very conservative number. The actual island of Iceland is quite a lot smaller than the full thing, there is a wide shelf, maybe some of that is glacial in origin like a massive underwater moraine, but that is still not going to explain all of it.
Even Hawaii is probably close to 1 million km3 if you include all of the current islands. Just the Big Island is 210,000 km3, and its abyssal footprint (the size of the base of the island) is not excessively bigger than the older islands, so they probably all reached that sort of size range at least for the 5 that are still above sea level.
Iceland is far larger than Hawaii, it is about as big as Olympus Mons at least in area, and maybe also just as tall if you consider the crust is 20 km thicker than average under Vatnajokull, so it would be that tall if gravity and the structural integrity of the crust were not important 🙂
Iceland is though definitely smaller than Kergualen, but then we will need to check back in another 15 million years to give them a fair age to age comparison, I think it would be a lot closer fight then 🙂
This is based on an old definition that is not often used, because it is not trivial to measure original flood basalt volumes. Area is much easier to get. The Icelandic Plateau is 350,000 km2 in area and has an average height above the sea floor of 1.7 km. That makes it 600,000 km3. You can argue about the average height: I think it is calculated from the average height of mid-oceanic ridges. In area, the Icelandic plateaus is about a quarter of the size of Kerguelen.
(The area includes the part below the sea. The island of Iceland itself is much smaller, of course)
Here is Iceland compared to Olympus Mons (and also Hawaii)
Actually, I was most surprised at how big Hawaii is compared to the other two, the volcanoes are huge but I always imagined it as being way smaller in area even being familiar with the dimensions. What I think is the true outer edge of Iceland is outlined, it is actually also bigger than I thought looking at them side by side.
I dont know how accurate the Hawaii scale is, I think it might be too small by some fraction based on how I moved the image on the map, but the 100 km marker is scaled correctly so it is close enough to be useful.
I think that volume is not something to ignore. It might be hard to measure the volume but in places where that is possible it is usually an important metric.
Many of the submarine LIP provinces are also calculated on the crustal thickness too not just elevation above seafloor. Also unlike most LIPs on land pretty much every oceanic LIP can be rather accurately measured as they are not eroded, so volumes for those are probably known and can be used as a legitimate definition.
I found there to be about 6 million km3 of material making up Iceland. That is based on the figure of 29 km being the average crust thickness of Iceland and that normal crust is 6-7 km thick, so Iceland is 22 km thicker. 6 million km3 is actually from the area of the outline on my picture above, times by 20 km, so it is probably a bit conservative for those dimensions but crust thickness data is only available for the on land part and not the shelf.
In any case, 6 million km3 is a lot bigger than most LIPs are, Siberian Traps are given as 2 million km3, up to 4 million. Deccan is up to 1.5 million. 6 million could be quite a high overestimation but even if Iceland was only 10 km thick it is still 3 million km3, and I have a lot more confidence that the whole plateau is at least 10 km thicker than the standard crust.
You are not comparing like to like. For the traps, you are taking the erupted volume, for Iceland you are taking the underlying crust. If you do that, much of the Andes should be considered as one because of the batholith. We need to stick to the same definition for the things that are compared. Yes, only 10% of Iceland’s magma erupts. If you use that to multiply the volume by a factor of 10 you need to do that everywhere.
That is what I did everywhere. I think a lot of oceanic LIPs are not equivalent to traps, including Iceland. There are trap events underwater too but I think assuming all of the plateaux are traps is not likely.
For all of the oceanic plateau LIPs I include the full thickness of their crust that is in excess of the thickness of normal crust, so whatever their thickness is minus 7 km more or less. While this doesnt represent the volume of eruption because it isnt all erupted material, I dont think it should be discounted from the volume because of that, because it still represents material that has left the mantle. Dike swarms under traps are the same thing, magma that didnt reach the surface but is still part of the LIP.
Point I am trying to make is that a lot of things that are presently referred to as LIPs would not count if Iceland is not on the list too. Like I have been saying LIP is defined in a bad way, it is used as if it is the same thing as a trap formation. But it really cant only mean the same thing as a trap formation, because a lot of LIPs that have been classified as such ever since the term was created, are not trap formations.
The Andes as a whole is not a volcanic structure so really cant count. I think though, there is a sensible case to include the silicic volcano complexes as borderline silicic LIPs. Same for the Taupo volcanics, might not quite compare to a traps event but a VEI 8 is still a VEI 8 and a lot of them have happened there in a relatively small area, and likely many more to come still. I dont know if plutonic structures are included in the category of LIPs though because it coudl be hard to distinguish them from cratonic granite. Wikipedia lists Large Plutonic Province as a thing though. I guess that means a trap formation would be a Large Volcanic province. Iceland would be somewhere in between maybe…
The whole discussion on the volumes of LIP’s relates to the general problems of mass balance right up to the scale of continental crust generation. This is particularly true of large silicic provinces such as Taupo and the Sierra Madre. A huge volume of silicic magma is necessary to balance out the intrusive/extrusive ratio and generate a huge volume of granitoid batholith. On top of this, a huge volume of mafic and ultramafic material, left over from the fractional crystallization of or partial melting of basalt/meta-basalt used to generate the silicic magma has to be taken into account.
To close the mass balance and generate proper continental crust, an enormous amount of ultramafic and or eclogitized mafic material left over after silicic magma generation must founder back into the mantle and be lost to the observable geological record. If this were not the case, silicic provinces would have huge negative bouguer gravity anomaly’s from these huge reservoirs of mafic/ultramafic materials. The fraction returned to the mantle is not available for direct volume measurement.
I think in most silicic LIPs, maybe even all of them, there is substantial basaltic intrusions right below the rhyolite. At least for the Taupo volcanics the calderas are heavily bimodal, both of the most recent eruptions at Okataina began with large basaltic intrusions. In the Kaharoa eruption this encountered rhyolite and made that erupt but the intrusion also created hundreds of hydrothermal craters to the southwest, maybe even with some of them erupting basalt. The 1886 eruption was basically the same except the dike didnt encounter any other magma on the way, so erupted directly. That is actually quite notable, maybe Tarawera has no more rhyolite under it, but it is unlikely there will be another eruption in the area for another few centuries so will be waiting a while to find out.
Yellowstone is also very bimodal, it erupts a mix of glassy rhyolite and tholeiitic plume basalt, the two complete opposite ends of the spectrum. It is I believe the only place plume basalt erupts on a continent today.
All of this mafic magma also stays in the crust, just like it would in any other setting, the reason it doesnt erupt much is because it cant mix with the rhyolite well and is more dense, like oil and water.
New article about the magma composition of the Fagradalsfjall eruption.
https://www.nature.com/articles/s41467-022-31348-7
Iceland is defentivly a LIP .. its an oceanic basalt plateau: But a rather slow one. Icelands gigantic igenous mass began forming around 20 million years ago .. and most of Iceland thats above ground have been formed since 14 million years, Iceland is productive… not not really the armageddon that are the LIPs that are fast enough to cause a mass extinction
Ontong Java Plateau is 100 million km3 and most of that was emplaced in much less than a million years. Siberian Traps was fast as well and as well as CAMP that was emplaced during a few pulses.. each lasting only a little more than a 100 thousand years
Real fast LIP flows Are catastrophic in scale
Hawaii is a LIP too .. volcanism on incredible scale but its prevented from forming a Mega – plateau by sitting on a fast moving seafloor that carries away the volcanic materials from the Hotspot
But indeed Hawaii is massive as well
Every single Hawaiian edifice dwarf any other volcanoes on Earth really
All other edifices are pretty much grains of salt when you place them infront of the Hawaiian edifices
Its the Fast moving pacific plate that prevents Hawaii forming an oceanic plateau.. had Hawaii been in Atlantic Ocean … thats 10 times slower moving then Big Island woud be 10 times bigger today 🙂
I dont think OJP was that fast, says it was over 3 million years on Wikipedia. If it was anything under a million years I think it would have sterilized the ocean…
Most of that volume was emplaced under a million years .. the ocean soaked up the CO2.
Is there an eruption at Suwanose-Jima? Or is this an Advisory exercise?
https://www.volcanodiscovery.com/suwanosejima/news/185129/Suwanose-jima-Volcano-Volcanic-Ash-Advisory-EXPLODED-AT-202207012316Z-VA-CLD-UNKNOWN-OBS-VA-DTG-0123.html
NASA Firms satellite shows nothing going on. The coordinates from FIRMS is [29.641, 129.714] while Google maps shows [29.642880, 129.715418] from google maps for the center of the volcano. The notice does NOT have the geodetic coordinates quite correct. I would say that this is a poor advisory.
I think that Suwanosejima is one of those places that is always erupting, not continuiusly but many times a year at least. So yes it is doing something 🙂 but seems not to be a place that erupts on a big scale.
New post is up! It is volcanic. It is miocene. And it is bizarre. Let Hector take you on a tour of Germany
https://www.volcanocafe.org/the-bizarre-miocene-volcanoes-of-germany-vogelsberg-urach-and-kaiserstuhl/
The Guardian also has an article that nicely ties in to this series:
https://www.theguardian.com/science/2022/jul/03/explosion-of-life-on-earth-linked-to-heavy-metal-act-at-planets-centre
I do wonder which act they are referring to. Motorhead?
Oh, that’s got to be Iron Maiden:
“When the world was virgin
Before the coming of man
Just a solemn witness
The beginning of the end
From a world of magma
To a cold rock face
The ascent of madness
And the human race”
This is rather speculative and based on thin data. It takes the most recent possible date for the formation of the core and ignores others, is based on measurement on one site, while other data indicates a stable field over a long time. The model for how to formation of the core would increase the field is also sketchy, although there are some existing models which suggest this. More data would be needed
I either wasn’t notified or missed the notification of your replies to my comment in the second post in this series regarding citation of sources, thus my belated reply. I’m not able to reply to your replies on my comment now, as comments are closed, so I am replying here:
1. If you are concerned about “readability”, then just include a bibliography and list of figures at the end of the article. Easy. This is still no excuse not to credit the hardworking scientists whose work you are using.
2. Your readers are likely extremely interested and willing to further research the topic, and giving them a bibliography would function as further reading material.
3. Your readers are likely not exactly the general population, having self-selected in finding and reading through articles on your blog, and they are probably more amenable to notes and citations than you think.
4. However, given the medium and audience, I would have to agree that inline citations are probably not the best way to go about it. It is true that some scientific articles are so chock-full-of citations that one must re-read sentences twice, even three times to understand them clearly. It’s not very ideal to lose your place while reading in order to check the works cited, and it’s also usually difficult to remember multiple authors names and the papers’ dates if you try to make a mental note and then check it at the end. Publications that opt for inline number-only citations, numbered in the order of appearance, often times listing multiple sources together (14, 15, 16) are the absolute worst. Most mass-market science books, which are also primarily concerned with “readability”, use footnotes are instead of citations. This eliminates the choppy reading experience, and many blog platforms have built-in or add-on support for footnotes, using two-way HTML anchors, which allow readers to jump to the footnote, and then directly back to the citation in the text, such that readers din’t need to lose their place. Still, many other mass market science books just make use of bibliographies, grouped by chapter, in the appendix of the book. In my opinion, this would be most appropriate for your blog.
5. Not including citations or a bibliography, when you use actual figures and findings from the published literature of working scientists is a detriment to your blog’s credibility. As I said before, there is no excuse not to include a bibliography and/or list of figures at the end of posts, at the very least. The tone of your articles is often “being talked down to by an eccentric tenured professor, who has been forced to teach first year students, and who has already had a couple drinks” (and that’s not meant as a dig—it can be entertaining, though honestly I believe sometimes you go a bit too far!). Look—it seems you want people to know that you know what you are talking about. What better way to show them, than to expose your sources and prove it? Unless, of course you don’t want people to see them, for some reason…
PS: this time, I made sure to check the “notify me” boxes.