It began on 24 May, 2015. Unannounced, unnoticed and unforeseen, in the middle of the night Wolf Volcano suddenly erupted. For all its fame, volcanoes on the Galapagos are not well monitored. After all, this is far from anywhere, so remote that not even the Polynesians found the islands. Its fame comes from its very isolation: life was given a very small palette here, but also a free hand where species could evolve without having to worry about Darwin’s fitness-for-life battle. Evolution experimented with its usual trial and error, but where in more competitive environments the errors would quickly have found their demise, here they had a second chance. Darwin noticed the range of rather similar finches in the Galapagos, each with a particular type of beak, but only realized its significance after a local pointed out to him that each particular kind would be found on only one island. In this isolated archipelago, even the different islands do not mix much. And so Darwin went on to discover evolution, the struggle for life and survival of the fittest, from an environment where that very struggle had been suppressed.
Darwin’s law has an in-build contradiction: survival of the fittest actually suppresses evolution. Darwinian evolution quickly gets to a situation where no further progress is possible. Take the shark: it has dominated the ocean for hundreds of millions of years. Its perfection leaves no room for a newcomer. It has an unfair advantage. To make progress, evolution first has to remove the fittest from the competition. Stephen Gould propose a ‘punctuated equilibrium’: a sudden change to the environment leaves previously optimised species unable to adjust and frees up room for improvement. Volcanic islands can provide this in an extreme way. They appear suddenly far from anywhere, without any species to provide competition, and only a few animals (or plants) find their way there. A world of opportunity opens up, and anyone can come up trumps. Evolution following a punctuated equilibrium cannot be predicted. In New Zealand, birds became rulers of all. In Guam (after some human intervention) it was the spiders. Australia (a more extreme example of an island) developed a neurotoxic tree. You couldn’t make it up.
In the Galapagos, far from the Madding crowd, evolution again avoided the struggle and worked a miracle. It did not go to quite the extremes of Australia. The most northerly penguins in the world have made their home here in the tropics, but they opted out from further evolution and remained faithful to their penguin life style. Finches evolved to fill some ecological niches unavailable in more crowded places. They aren’t as eye catching as say a moa would have been, but they managed some unexpected adaptations. Who would have imagined a vampire finch, making its living by stealing blood from other birds? Miracles are not easy to notice. In the Galapagos, it required the eye of Darwin to see the wonder.
But the Galapagos is not just a paradise for evolutionary biology. Its volcanoes are also worth studying. For they too evolve, with hidden wonders.
The islands
The Galapagos Archipelago contains 13 significant islands, all volcanic. The currently active volcanoes are found on the western islands. These islands are also much larger, consistent with a frequent supply of new island-building material. In Isabela 6 volcanoes (one partly eroded by the sea) merged into one island; Fernandina with its single volcano may soon join. All 7 volcanoes have a similar shape, looking like a an upturned soup bowl, with a large central caldera (that part is not from the soup bowl), a flat rim typically a few hundred meters wide, and beyond this rim a steep side (up to 35 degrees) which becomes much shallower further out. Eruptions are effusive, and the calderas have formed by collapse rather than explosively.
There is a clear progression among the islands. The eastern islands are older, less volcanically active and less basaltic. The oldest islands in the Galapagos are around 3 million years old, whilst the youngest island, Fernandina in the west, may be less than 100,000 years old. The progression in age and activity fits the movement of the underlying plate, which is running to the southeast at around 5cm/year, or 50 km in a million years. The Archipelago is around 200 km long in the direction of the plate movement. This corresponds to 4 million years of plate movement, approximately the age of the oldest islands. Thus, all islands first formed at roughly the same place. This is exactly what is expected from a stationary hot spot: underneath Galapagos is a region of hot mantle, which causes melt, while the plate moves over this region. Every now and then, the molten magma punctures the overlying crust and builds a volcano. After a while the volcano’s conduit has moved inconveniently far from the source; the magma now reconsiders its fidelity, abandons the old volcano and punctures a new hole. A new volcanic island rises, and the abandoned island slowly erodes and sinks below the sea, taking its unique biological cargo with it.
Two of the islands are much further north, 100 kilometer from the rest of the archipelago. These are mainly seamounts with a top above water and are called Wolf island and Darwin island. Confusingly, Wolf volcano and Darwin volcano are not on those islands, but are on Isabela.
The Galapagos are sitting on a large plateau which rises 3 kilometers above the surrounding seafloor. This also is a strong indication of a hot region underneath. The hot mantle and magma have a lower density than the colder material of the surrounding oceanic plate and underlying mantle, causing the sea floor to bulge and rise. The same effect is seen in Iceland which has risen so far that the entire bulge ended up above sea level.
The most active volcanoes of the Galapagos are Fernandina, Cerro Azul and Wolf. They erupt through several types of fissure: radial fissures along the flank, a ring fissure around the caldera, and fissures inside the caldera. Eruptions seem to alternate between the the flank and the ring. Fernandina erupted through a flank fissure in 1995, from the ring fissure in 2005, and again from a flank fissure in 2009. Wolf had a flank fissure eruption in 1982, and a ring fissure one in 2015. Lava flows tend to be quite short. The rounded, steep shape of the volcanoes were build by short flows from the ring fissures. Both volcanoes have two different magma chambers. The eruptions are fed from a shallow chamber underneath the summit, at a depth of 3 kilometer below the summit. A deeper chamber at around 7 kilometer below the summit inflates before an eruption, and feeds the upper chamber.
The volcano and the tortoise
Wolf volcano is 1700 meter high at the rim of the caldera. The caldera is 700 meters deep and 5 kilometer across. The equator runs throught the southern half of the caldera. Since 1797 the volcano has erupted about once per twenty years on average. The last eruption in the 20th century was in 1982. A decade later the floor of the caldera started to inflated, first by 1.5 cm per year, later by 3 cm per year. This culminated with the 2015 eruption. The eruption began on 24 May, 10 minutes to midnight local time (it is usually given as May 25 which was the date in GMT) when a sudden explosion sent a gas plume (but only a little ash) 15 kilometers high into the atmosphere. Lava erupted on the ring fissure along the southeastern caldera rim. The erupting rift was 800 meter long with 100-meter high lava fountains. The erupting fissure was bisected by the equator: an eruption at a latitude of exactly zero must be rare! If it had occurred a month later, both the Sun and the Moon would have been directly overhead as well (although not simultaneously) – the pseudoscience community would have had a field day. Luckily they didn’t. Later the fissure extended further into the northern hemisphere by another kilometer. The lava reached the sea within three days.
But that was as far as it got. The eruption ended on 2 June (a full Moon), after only 8 days. We have become accustomed to long-lasting fissure eruptions, after Holuhraun, Leilani, Fagradalsfjall and Cumbre Vieja. This was not like that. Was this because it was fed through a shallow magma chamber which lacked the volume for something longer? Would a direct connection to a deeper magma chamber have allowed for something more? The eruption started fast and furious, but rather quickly declined and shut down again.

The 2015 lava flows are shown in red. Grey dashed lines are fissures, black for those involved in the eruption. Light blue are fissures involved in the 1982 eruption, the most recent one before 2015. Source: “The 2015 Wolf volcano (Galápagos) eruption studied using Sentinel-1 and ALOS-2 data” by Wenbin Xu et al Geophysics Research Letters, 2016 https://doi.org/10.1002/2016GL069820
On or around 11 June (the date is not precisely known) activity resumed, but now inside the caldera. It ended exactly 1 month later, 11 July 2015, by which time about half the caldera floor had been covered with lava. By the end, two lava fields had formed on the flank covering 17 km2, and one in the caldera covering 3.5 km2. The total lava volume is just under 0.1 km3. This is larger than average for Wolf volcano: the 1982 eruption was smaller, at 0.05 km3.
I wish I could state that no animals had been harmed in the making of this eruption. I can’t. Wolf Volcano has its own subspecies of giant tortoise, an animal not well equipped to run away from lava. Even the Lurking maxim, ‘don’t be there’, would require significant advance warning. However, the tortoises live on the vegetated western and the northern slopes of the volcano. Eruptions are mostly toward the south and east, where they have removed the vegetation and replaced the landscape with a desolate lavascape. The tortoises got the message, or at least those tortoises being evolutionary awarded with an attraction to those fields of cold lava would have found themselves in pole position for a Darwin award. But if the volcano ever changes sides, the tortoises might find themselves in a punctuated equilibrium with plenty of room at the top.
In fact, it appears exactly this has happen before in the Galapagos. There are five complete volcanoes on Isabela. Each has a different species of giant tortoise living on its flanks. One of these species, which happens to be the most numerous, lives on the slope of Alcedo volcano. It is the one that all the tourists get to see, being closer to the settlement on Isabela. This particular species has remarkably little genetic diversity. It appears that there was a severe population bottleneck around 90,000 years ago. And Alcedo indeed had a significant explosive eruption 100,000 years ago which covered the slopes in pumice. Very few tortoises may have survived this – possibly (models say) only a single female was left to continue the breed.
The caldera wall of Wolf, 700 meter deep, cuts through lava flows of the past. These older flows are not visible on the flanks: because erosion here is slow, new flows cover up the past faster than that erosion can reveal the ones who went before. The caldera wall shows two different types of flows. One type is similar to the recent caldera flows: a’a flows between 2 and 5 meters thick, traceable over several kilometers. The other type shows shorter, thinner (0.5-1 meter) pahoehoe flows. Based on recent behaviour, the pahoehoe flows are though to be the ones that have build up the cone, emanating from the ring fissure at the top. The a’a flows are those that filled the caldera. The 1982 eruption also formed such an a’a flow, emanating from the bottom of the wall. These a’a flows are only seen more than 450 meters below the rim, suggesting that either the caldera was always at least this deep, or that it did not have these flows during times when it was shallower. Avalanche deposits on the caldera floor, partly covered by the recent lava, suggests that the caldera wall is not fully stable and is prone to collapse.
An interesting finding is that the caldera wall shows evidence for dikes that run under an angle to the wall, but these do not connect to the ring fault. This seems common in Galapagos volcanoes: dikes in the caldera stay inside the ring fissure.
The magma in Wolf volcano is basaltic. The Galapagos has a range of basaltic magmas. Near the hot spot, which is probably currently centred near Fernandina, the magma has a composition that indicates an origin in the deeper mantle. Volcanoes further from the centre of the hot spot have magma that is more similar to mid-ocean-ridge basalts (abbreviated MORB), as found at the nearby Galapagos Spreading Centre. Wolf is situated at the northern edge of the hot spot, and it has magma with isotopic ratios similar to that of the spreading centre. Wolf has even been called the most MORB-like intraplate volcano in the world. That suggests that it derives its magma solely from melting ocean floor and upper mantle. It may gets its heat from the hot spot, but it has to make its own magma. The composition indicates low partial melting of a few per cent. In spite of this, Wolf is frequently active with an apparent eruption rate of 0.3 km3/century.
The cause of the hot spot is hotly debated. At the current time, the hot spot is not particularly large. It is located in a very complex region, on a micoplate near a spreading centre. This makes it difficult to study the details. The hot spot has left a chain of defunct islands stretching towards South America where they subduct below the Andes. The length of the track shows that the hot spot is at least 20 million years old. It could be much older. Along the coast of South and Central America lies a feature called the Caribbean plateau. The accretion of this plateau, perhaps 70 million years ago, may be the cause of the very thick continental crust underneath the Andes. The origin of the Caribbean plateau origin is controversial. It may be a remnant of a flood basalt, carried there by the subducting plate. Alternatively, it is also suggested to be the accumulation of all the old Galapagos sea mounts and ocean floor lavas swept up by the subduction zone. In either case, it is linked to the Galapagos hot spot, and this is confirmed by the fact that it has the same isotopic and chemical composition as the Galapagos. One part of this basalt, at the Nicoya peninsula in Nicaragua, is dated to 140 million years old. That would imply that the hot spot is at least that old.
(Why is it present in both South and in Central America? The Galapagos is located close to the spreading centre. Over time, some of the islands have found themselves on the other plate, and taken towards Central America.)
The rocks of the Caribbean plateau are mafic to ultramafic (komatiite) and require high mantle melting temperature of 1550C. The current Galapagos lavas have a melting temperature of around 1400 C. If the two have the same origin, it implies that the hot spot has cooled by about 1 C per million years. Temperatures as high as 1500C are rare, and require a mantle plume. In this model, the plume has been cooling over perhaps the last hundred million years.
The Mermaid and the plume
But is there evidence for the existence of a plume? This requires seismological mapping of the mantle, using information from earthquakes. But seismographs are normally placed on solid ground and this leaves little room for them in the deep Pacific. There are about 11,000 reporting stations on land, but only 500 in the open ocean. Subduction zones tend to be close to the coast and are well mapped. Hot spots are mostly below oceanic plates and they have much less data. MERMAIDs provide a possible solution.
The acronym, somewhat awkwardly, stands for ‘Mobile Earthquake Recording in Marine Areas by Independent Divers’. These are instruments which float freely at a depth of 1500 meters, and come to the surface to report their data by satellite. Their life time is limited to about a year because of the battery. When a P-wave from an earthquake travels through the ocean floor, it causes an acoustic signal in the water. The MERMAID picks up this sound wave. When it detects one, it records the arrival time, surfaces to report it, and dives again to resume duty. The report is compared to the earthquake catalogue to see whether it relates to a true event. About half were confirmed to be earthquakes, mainly M6 and above. The MERMAID system was tested in the Galapagos area in 2014 and 2015, using 9 such instruments. The result of this experiment were reported in a paper in 2019, Nolet et al. Scientific Reports, 9, Article number 1326 https://www.nature.com/articles/s41598-018-36835-w
The results from Nolet et al. showed a region in the mantle (in red) where the P-waves have a low velocity. This indicates a high temperature: a plume. This plume extends to 1900 km depth. It does not go down to the core, but it extends well below the 400km and 600 km layers which separate the upper and lower mantle. The plume comes up underneath the spreading centre, at a location north of the Galapagos, approximately where the Cocos ridge crosses the spreading centre. Near the surface, the spreading motion of the plate deflects the hot region southwestward, towards the Galapagos.
The information from the MERMAIDS is not conclusive and needs more work, but it suggests there is indeed a deep mantle plume here extending about halfway to the core.
So what exactly is going on? If we assume that the data is correct, then the plume is located close to where the spreading centre jumps southward, with a short transform fault which connects the segments. The plume comes up at around 91 degrees west, 2 degrees north, which puts it just to the left of the transform segment, and close to the northern spreading centre.
What follows is my own speculation: be aware!
The Cocos ridge and the Carnegie ridge both arise from the Galapagos hot spot, going in different directions on different plates. The two ridges are 20 million years old: therefore, the hot spot has been on the spreading centre for at least this long. But the Cocos ridge has a gap near the spreading centre, and the Carnegie ridge has a gap midway. It appears that the hot spot wasn’t exactly on the spreading centre but spend time on either side, forming each ridge almost in alternation.
The hot spot is supposedly not moving, as it is tied to the deeper mantle. However, spreading centres do move. They are like paper being torn by the two sides being pulled in opposite directions. The paper can tear at any place. Now imagine that glue is put in the tear. The paper may decide to tear somewhere else. Oceanic plates are like that: they tear, but the tears can heal and reform somewhere else. You can see this in maps of mid-oceanic ridges where different parts do not line up and require perpendicular transform faults to connect them. Iceland is an example of a region where the tear moves rather frequently. But even without jumps, the plate on which the spreading centre forms is itself not stationary. The centre moves with the average movement of the plate – often westward.
The Galapagos hot spot thus has found itself on different sides of the spreading centre at different times. At the moment (perhaps since the past 5 million years) it is on the south side. The spreading centre mainly pulls up magma from the local mantle, not from the plume, although it has however been suggested that some magma from the plume makes it to some parts of the spreading centre through faults.
The Galapagos plume now hits an asthenosphere and lithosphere that is moving away from the spreading centre, and it gets pulled along. A hot plume can develop a wide, mushroom-like head. This widening is caused by the lower viscosity of the hot plume. But the Galapagos plume isn’t that hot any more, and it hits a lithosphere which is itself warmed by the spreading centre. So the plume stays narrow as it bends towards the Galapagos; it surfaces in only a part of the Archipelago. Wolf Volcano misses the main plume but captures the edge of the plume, warmed by thermal diffusion. This melts the oceanic crust, and feeds its eruptions.
Here ends the speculation. As all speculation, it should be taken with great caution.
The mantle plume material has been shown to travel much further with the plate. Recent studies (published only a few weeks ago: https://www.sciencedaily.com/releases/2021/11/211122135425.htm) have found that volcanic rocks in Panama have high amounts of 3He, indicating a link to the deep mantle. The elements and isotopes indicate that the material has the same source as the Galapagos. The mantle plume material appears to have been carried below the Cocos plate, and reached Central America, or at least it did so 10 or 20 million years ago. The authors call it a ‘mantle wind’. It is in fact plume material being captured by the moving plate.
The volcano: hidden diversity
As mentioned, the Galapagos volcanoes in different parts of the island bring up different magmas. The Galapagos Spreading Centre also shows somewhat different magmas close to and further away from the Galapagos Archipelago. Within the islands, there is a core region with plume-like magma, and a half ring around it where the volcanoes are mixed, or more mid-oceanic like. This behaviour is also seen along the trail left by the hot spot: the central line is plume like, the outer lines are oceanic-crust like. This behaviour has been consistent for at least 10 or 20 million years. It has been argued that this structure is inherent in the plume itself, and that the material brought up from the deep already has different compositions in the core of the plume and along the outer edge. The data only shows what comes up, not how the magma came to be. There is more research to be done.
But research has found more diversity in the Galapagos. Even in a single volcano, there appears to be more than one type of magma. This is even so when all the eruptions are of one type of magma only.
The story begins underground. As magma ascends, it evolves. There will be some crystallization as the magma cools, some crust may get incorporated, and some mixing with older magma may happen. There are volcanoes that can erupt more than one type of lava, but many don’t. It is actually surprising that lavas from different eruptions from the same volcano tend to be so similar, in view of everything that can happen to it on its journey. Perhaps all magma travels up in exactly the same way, through a single and uniform large magma chamber, or perhaps any underground reservoirs are fully flushed before each eruption – but both possibilities seem somewhat unlikely in view of what we know about volcanic plumbing.
In the Galapagos, the volcanoes on Fernandina and Isabela (including Wolf volcano) each produce very uniform basalt. Different eruptions from the same volcano produce lava that has a storage temperature that is the same within 30C, around 1100C. The storage temperature is the temperature at which the crystallization happened. (It is not the original melt temperature of the magma which is much higher.) The lava here contains crystals of olivine and of other minerals. Each mineral has a different temperature at which crystallization happens. Therefore, which crystals are seen provides a good underground thermometer. Volcanoes on the other islands, further from the hot spot, show more diversity between eruptions. This has led to suggestions that in fact the hot spot is responsible: volcanoes become uniform if the heat flow and magma input are high enough to create a stable magma layer, which acts as a large buffer for any new magma. Volcanoes further away lack this. The ‘magma zone’ is in fact a mush layer, a mix of solid and liquid material which itself cannot erupt but which interacts with any ascending magmas.
The 2015 eruption of Wolf volcanoes produced extensive lava flows. The earliest, more explosive phase of the eruption also produced some tephra. Analysis of the lava and tephra has shown that about 10% of the material consist of crystals that had formed in the magma, at a temperature of 1160C. When these crystals were analyzed (Stock et al, 2020, Nature Communications 11, Article number 3767), it gave a bit of a surprise. Although the lavas had been remarkably uniform in composition, the crystals from this one eruption were not. As an example, the amount of calcium in plagioclase crystals varied by a factor of 2 between samples. A study of the 1968 lava from Fernandina showed a similar (but different) diversity. Especially the interior of the crystals were highly evolved. They had spend a long time in storage, possibly millennia, but there had been different storage units with different magmas. The crystals show little ‘zoning’ (changing composition with depth in the crystal) other than the rim, which suggests that each storage unit was itself stable. The changes in the outer rim were caused by interaction with the erupting magma.

From Stock et al. 2020. Plagioclase crystals in lava and tephra samples from the 2015 Wolf eruption are shown as blue squares, nodule samples from the 1968 Fernandina eruption are shown as red diamonds and lava samples from historic Fernandina eruptions are indicated by open diamonds. The horizontal axis shows the percentage of calcium compared to Na+CA+K.
The storage contained magma with silicic compositions. (As magma crystallizes, the SiO2 fraction of the remaining liquid increases. This evolves the magma from a basalt (around 50% SiO2) to an andesite (60%): it becomes silicic.) But Wolf has never erupted silicic magma, as far as we know. The same was found for Fernandina: the crystals show the presence of evolved magmas that have themselves not erupted. These are basaltic volcanoes – but with silicic magma in store.
The result shows that Wolf volcano has a series of long-term magma storage units where the magma has a range of evolved compositions. These storage units were accessed during the 2015 eruption, and the crystals carried up with the new, more primitive magma. The crystals and magma were not in equilibrium: they came from different places. The storage had happened mostly at a depth of about 7 km, but the initial explosion ejected some crystals that were stored at around 1 km depth. The storage was initially filled with primitive magma, but over time became evolved and crystal-rich. During this process, these stored magmas had become viscous and therefore un-inclined to erupt.
This happy state of affairs became interrupted during the eruption. New magma ascended, entered some of the storage units and mobilized the stale magma. As phrased by Gould, the happy equilibrium was punctuated. About 10% of the lava erupted by Wolf comes from these old chambers – not enough to change the composition. But the crystals in the lava mostly come from this 10%.
Is there any evidence for this, apart from the crystals? At Fernandina, some submarine lava flows (off-shore, obviously) from flank eruptions show a different, more evolved composition. They can be fitted with 50% contribution from the evolved magma storage, coming only from the magma chambers at 7 km depth.
The cartoon above (also from Stock et al. 2020) illustrates the picture that arises from this. Typically, the evolved magmas are heavily outnumbered by the more primitive magmas that drive the eruption. In the lavas we only see these primitive, basaltic magmas. But there is in fact a silicic mush present. As long as the magma formation rate is larger than around 10-4 km3/yr, the primitive magmas will dominate. Wolf and Fernandina both have rates that are a few times higher than this. But if the melt rate would drop below this value, as has already happened in the eastern Archipelago, the eruptions can become more heterogeneous. Now, a basaltic volcano could suddenly have a silicic eruption.
And the equilibrium is punctuated when the volcano may suddenly explode. The Alcedo tortoise found out the hard way. Never trust a volcano.
The tortoise and the volcano
The Galapagos is a place where evolution has rapidly diversified a few refugee species. It lacks the extremes: by and large, the species look fairly normal, but with very diverse specialisations. But where else can you find a vampire finch? And the volcanoes too hide a surprising diversity. Like the animals, the magmas have traveled a long way to get here: 1900 km from the deeper mantle (but not the core-mantle boundary which is much deeper – thereby making both the ‘shallow and the ’deep’ hot spotters unhappy). The magma traveled twice the distance from the Galapagos to South America. And like the animals, the volcanoes diversified. The volcanoes may look similar, and normal. But they hide diversity both above and below ground. And the bottom line is a warning: be prepared. When equilibrium is lost, a basaltic, effusive volcano may suddenly change and explode in a burst of volcanic evolution. Alcedo did it, and almost wiped out the tortoise that depended on it.
Wrangellia
There is one more thing about the Galapagos. Remember the million years of rain? It was caused by the Wrangellia flood basalt. But which hot spot was responsible? It has been suggested that the only one known in the area where it happened, is the Galapagos plume. The isotopic ratios and temperatures of its flood basalt is remarkably similar to that of the Caribbean plateau. It would require that the hot spot is over 200 million years old. There is no evidence that hot spots live that long – but we don’t know that they don’t either.
If this is true, then the Galapagos plume is responsible for more than just a vampire finch. For the million years of rain lies at the origin of both the dinosaurs and the mammals. When the struggle for life is suspended, evolution can go far. Stephen Gould would have approved.
Albert, December 2021
I guess Jesper will show up again after lunch. The big mass east of Mayotte must have robbed his sleep. 🙂
For some people here who stumble into the same problem I had:
Once upon a time there was the KT-Boundary, K for Kreide=Cretacious, T for Tertiary. Back then there was the early, middle and late Cretacious like the early, middle and late Jurassic/Triassic. That was brillant and understandable.
Then geologists or paleontologists or both introduced new nomenclature. So now it’s called the K-PG boundary, 66 to 65 Ma. The Paleogene spanned the time from 65 Ma to 23 Ma, after 23 Ma the Neogene started. Then this was split up futher into the Paleocene to 55 Ma, the Eocene to 34 Ma and the Oligocene to 23 Ma. The Neogene was split up into the Miocene, the Pliocene and the Quaternary (we are living in it).
I ended up getting myself a table printed lying next to my desk. The Pleistocene isn’t in it. So here is the Pleistocene:
“The Pliocene and Pleistocene epochs are commonly combined and referred to as the Plio-Pleistocene because of their short duration in comparison to previous epochs, and also because the events that took place during these epochs are very closely associated. The Pliocene spans the interval 5.3 – 2.6 MYA and the Pleistocene 2.6-0.01 MYA.”
https://research.amnh.org/paleontology/perissodactyl/concepts/deep-time/plio-pleistocene
MYA was also changed into Ma. I complained to my daughter aboit it who has studied Classics. She said it must mean Megaannum ago. I still prefer the Mya. That obsession with renaming is annoying.
It’s just useful to have such a table. And if no table is found it might be useful to write it down for oneself with the help of a search machine.
But maybe other people do not have such a problem, and I am just plain stupid, who knows.
You are possibly mixing up different levels of the nested time elements of the stratigraphic column. The Pliestocene is nested with the Holocene in the Quaternary; it is definitely distinct from the Pliocene.
The International Commission on Stratigraphy is your go-to reference here.
https://stratigraphy.org/chart
Yes, of course. The Pliocene was before the Pleistocene and is the last phase of the Tertiary, and of course, the Pleistocene is the first phase of the Quarternary. All together is called Cenozoic.
Thanks for the reference, but I will do better with my table with all the phases and expressions next to each other. It’s sane to look at a piece of paper or a book sometimes, at least for me.
Mount Damavand (Chad) is beautiful and part of the Volcanic Seven Summits.
https://en.wikipedia.org/wiki/Volcanic_Seven_Summits
Too beautiful to erupt and part of Persian mythology.
Thank you Chad, looked at lots of pics, also from a ski tour. It’s a beautiful landscape.
A fumarole here:
Better not erupt:
“Fereydun strikes Zahhāk down with his ox-headed mace, but does not kill him; on the advice of an angel, he binds Zahhāk and imprisons him in a cave underneath Mount Damāvand. Fereydun binds Zahhāk with a lion’s pelt tied to great nails fixed into the walls of the cavern, where Zahhāk will remain until the end of the world.”
So, the guy, in older sources a three-headed dragon, is in a cave under Mount Damavand. Every beautuful strato has its own legend:
https://en.wikipedia.org/wiki/Zahhak
After this I’ll take a break from my flow of evening and morning comments. Hope siome were interesting. As two the seven Volcanic summits I discovered that Elbrus is on the Greater Caucasian fault system, Damavand on the Lesser Caucasian fault system, both running parallel to each other.
As I very much liked Albert’s piece about the North Anatolian Fault, I’d like to suggest a second part centred on the area north an east of Turkey, with Elbrus and Damavand close to the Black Sea and Caspian Sea for next year, if interesting for Albert, of course. Literature:
https://www.researchgate.net/figure/Active-tectonics-north-of-the-Arabian-plate-from-Avagyan-et-al-2005-1-Major_fig1_258391428
corr: as to the seven
Hi, excuse me for the OUT, but I would like to know one thing, and I do not know who to ask, except you.
Meanwhile, assuming we don’t know the exact dynamics of a “supereruption”, I would know if a supereruption triggers single giant explosions or, roughly speaking, the rhyolite is emitted less violently in a lot of time (weeks, months) depending by the eruption. When we speak in terms of MEGATONS or GIGATONS in supereruptions, that measure is, in some way, a real value of energy or power of explosions? Are there explosions of GIGATONS in energy in supereruptions?
A friend in QUORA, confronting the power of a supereruption with Krakatoa (150-200 Megatons in the explosion at 10.02 a.m. on the 27nd of August), sustains that if a VEI-6 produced those effects/explosions reported historically, what to say for single giant explosions during supereruptions? He reported that they could flat entire nearby forests or destroy mountains, like the energy released in a giant asteroid impact, so GIGATONS are a REAL value for a supereruption. He sustains this for a documentary in which was explained that shockwaves/soundwaves flatted entire forests in Indonesia and India and because range mountains nearby giant calderas producing supereruptions disappears after the event, it means, for shockwaves; I’ve shown my doubts saying that the range mountains disappear could be explained well enough for the subsequent collapse of surface after a supereruption, BUT WHO KNOWS…. Question is: rhyolite is emitted with giant explosions (in GIGATONS) or with pyroclastic flows, collapses, or both?
What do you say? Someone could put please studies, articles about?
First of all, Krakatau was not a supereruption, not even by a longshot.
Secondly I would suggest you read this article about a very small supereruption:
https://www.volcanocafe.org/the-great-american-volcano-aniakchak/
Let us just say that not being around is a very good idea when it comes to VEI7+ eruptions.
I’d like to add this, maybe useful:
https://www.volcanocafe.org/calderas/
amazing picture of Katmai.
and also this:
https://www.volcanocafe.org/future-calderas/
A caldera-forming collapse like Aniakchak (Carl’s piece) or Thera (in the caldera piece) would obviously be a very sudden event like an impact. I think that’s what you wanted to know. That’s why there is a certain fear of Yellowstone due to Yellowstone’s history (caldera piece).
A Large Igneous Province like the Siberian traps, assumed responsable for the Permian-Triassic extinction, is said to have happened during one million years, similar estimates for the Deccan traps. That wouldn’t take down forests right away, but cause a climate change due to the gasses.
If you study all three pieces you get an impression. I did at least.
Explosive eruptions can flatten forests but not because of shockwaves. The pyroclastic flows that travel at high speeds away from the location of a large volcanic explosion knock down the trees and can even erode into the ground. But they are currents of dense material not shockwaves.
This article is great in describing the effects of a large volcanic explosion, in Merapi 2010, including the blow-down trees, but unfortunately it is not open access:
https://www.sciencedirect.com/science/article/abs/pii/S0377027313000176
I found this article doing a quick search, not sure if it might be what you are looking for:
https://agupubs.onlinelibrary.wiley.com/doi/pdf/10.1029/2008JB005848
Hello, Ector, thanks to you and everyone for links and everything. Yeah, I know about pyroclastic flows flatting forests.
Let’s say that my question is if supereruptions produce large explosions for which speakings of GIGATONS of TNT have sense, surely as far as we know about this type of eruptions.
My friend in Quora heard in that documentary of entire forests flatted in India (continental level) for sound/shockwaves (nothing pyroclastic flows, propelling thereselves for “only” hundred of kms); maybe some documentary can exaggerate, but it’s natural speaking of GIGATONS of power hearding such a thing, considering, for example, Krakatoa itself, a little thing compared to a supereruption, but it should be proud for its 200 Megatons. I red in the past the Aniakchak article, but remains that its eruption is different by a giant calderas erupting because was a magnific lateral blast. Here to say, if a supereruption produce similar single giant explosions of GIGATONS, as far as we know.
Meanwhile, I’ll read your articles, for which I thank you once again
You need to tell us what unit you are using. Megaton of what? Engineers might quote it in TNT, physicists will quote it in Joule. Without that information, this is an unanswerable question.
But to give an idea, to fully fragment 1m3 of rock by blasting requires roughly 10 MJ. A decent explosion (say Tambora) evacuated 50 km3 in one bang. That is 5 times 10^17 J. And that is just the fragmentation. Now you still need to energy to throw it out, to create the sound wave, etc.
Krakatau was a fairly large but not exceptional eruption. Tambora was quite a bit larger, and yes, it was mainly a single explosion. There were no known survivors within 20 km of the site.
Megaton of TNT, for measuring explosive energy
1ton TNT is 4 giga-joule. Tambora would have been something like 10^8 times that for fragmenting the rock, so 100 megaton TNT just for that. So yes, I would think that the largest single volcanic explosions are in the gigaton TNT range. There are of course much bigger explosions. The dinosaurs were killed by a 10-km asteroid. The kinetic energy of that would be something like 5 10^22 J, and all of that went into a single explosion. Say 10 tera-ton TNT
Do you say that for Tambora there was a single explosion in the order of hundreds of megatons to evacuate 50 km3? In effect, its explosions have been heard up to 2000 kms approximately, according to reports. But, if we consider the possibility of single explosions of such a power in “normal” volcanoes, what for a supereruption?
Even if in this article it is sustained that Toba evacuated its material “slowly”: https://www.volcanocafe.org/the-toba-supereruption/
Yes, Toba was different and not strongly explosive. VEI8 (super eruptions) are not like the big explosions. https://www.volcanocafe.org/power-of-the-past-25-super-eruptions/. Tambora, and similar events like Rinjani and Ilopango (all VEI7) were big bangs
In supereruptions you may get a series of Tambora size explosions. If I remember right the Hatepe VEI 6 eruption of Taupo had a massive explosion at the end making an large ignimbrite. The Oruanui VEI 8 eruption of Taupo was many Hatepe-like explosions happening one after another.
If you look the ignimbrites are the same size despite Oruanui being over 10 times bigger in volume. That’s cause the intensity was really the same, Oruanui was simply was made of more blasts than Hatepe:
To Microwave: VD has a nice pic of Mount Etna from Tuesday. With some snow on the top, frame for the lava fountain.
Thank you Denaliwatch!
I particularly like the picture called “Last night’s lava flow in the Valle del Bove (image: Giuseppe Distefano / Marco Restivo / EtnaWalk / facebook)”.
The yellow hot looking lava flow within the snowy Etna is incredible!
How does the supply of Etna compare to Iceland and Hawaii anyways?
New post is up! Hector takes Hawai’i apart
https://www.volcanocafe.org/kilauea-iii-rifts-under-hawaii/
Albert and Héctor, I’ve found two recent articles about Toba supereruption, according to them Toba could have emitted up to 10^11 kg/s of materia, clearly very explosive; this in opposition to a “quiet” Toba supereruption, according to the article Albert linked on this theme. The first author is Italian (Antonio Costa) and one study is the same estimating for first 13200 km3 for Toba.
I’ll put relative links
That is indeed the expected eruption rate. It was 2800 km3 in nine days, or around 10^10 kg/s on average. A ten time higher rate over say the first day is entirely expected. It erupted on the entire ring of the caldera, perhaps 250 km long. Think fire fountains, where the heat carries the ash and gas high up into the stratosphere. AN explosive eruption would have had a far higher peak eruption rate. It probably started with an explosive opening of the fissure, but that was not the main event
Hi Albert, thanks for answering. Here the articles:
https://www.frontiersin.org/articles/10.3389/feart.2014.00016/full#h9
https://www.nature.com/articles/s41467-018-02901-0#:~:text=%20Understanding%20the%20plume%20dynamics%20of%20explosive%20super-eruptions,order%20to%20avoid%20making%20unrealistic%20assumptions%2C…%20More%20
Yes, this team has been arguing for more extreme eruption parameters, but not necessarily a single explosion. They argue for 15-hour duration. (You were asking about single explosions.) I don’t quite recall where the evidence for nine days came from, but it may be from wind directions during the eruption (as traced by the ash distribution). The second paper you mention has a problem: they need to set one of the parameters to an inconsistent value to make the results fit. That suggests one of their assumptions may be wrong. We think that the eruption column reached over 40 km because of the sulphur record in the antarctic ice cores. However, that does not require a single explosion: just the heat from an event this size can create gigantic convection. Ash was distributed by the tropospheric wind patterns: there is no indication that significant ash reached the higher stratosphere, and certainly not the mesosphere, as that would have given a much symmetric ash distribution. Eastern Indonesia was hardly affected while India had a decent ash fall: that means wind. Gas (sulphur) could reach higher but ash fell out of the eruption column. This was not an instantaneous explosion.
Yeah, I asked for large explosions (as single blasts) during supereruptions, independently by their lasting. For example, if in a supereruption lasted 9 days there could have been a blast or more than one of, for example, 400 or 500 megatons and over. Another interesting question could be if, after the ring opening, the climax phases started with such blasts, even if, generally, rhyolite was emitted slowly
Not an easy question to answer. There may have been many VEI6’s within Toba and we would not know. Taupo is probably a better case for a very large explosion. Or perhaps more than one.
Ok, Albert, thank you very much!!!
La palma LP03 maintain deformation.
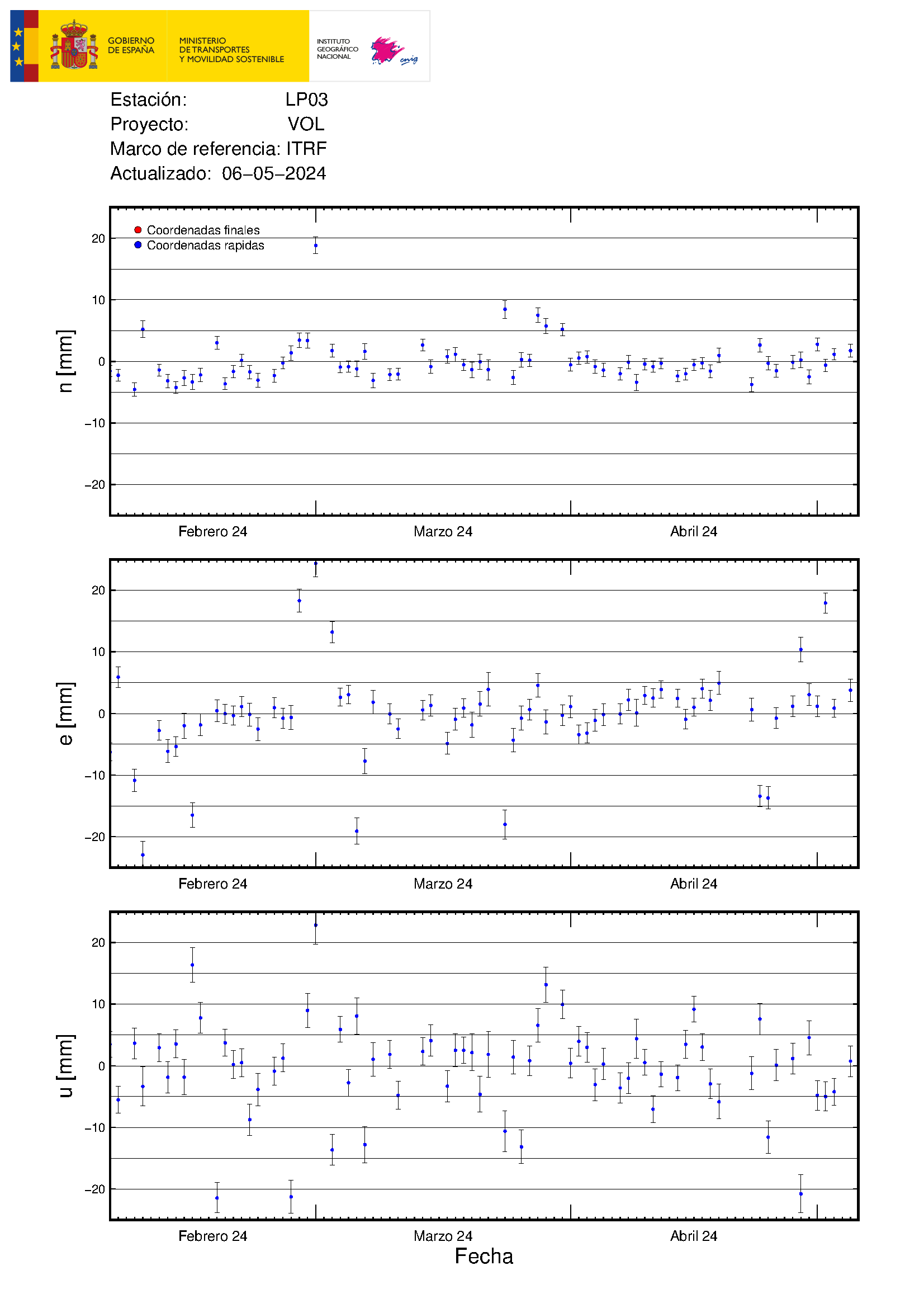
It may be better to post this under the current article. Not many people check past posts for new comments